Ardem Patapoutian
Biographical
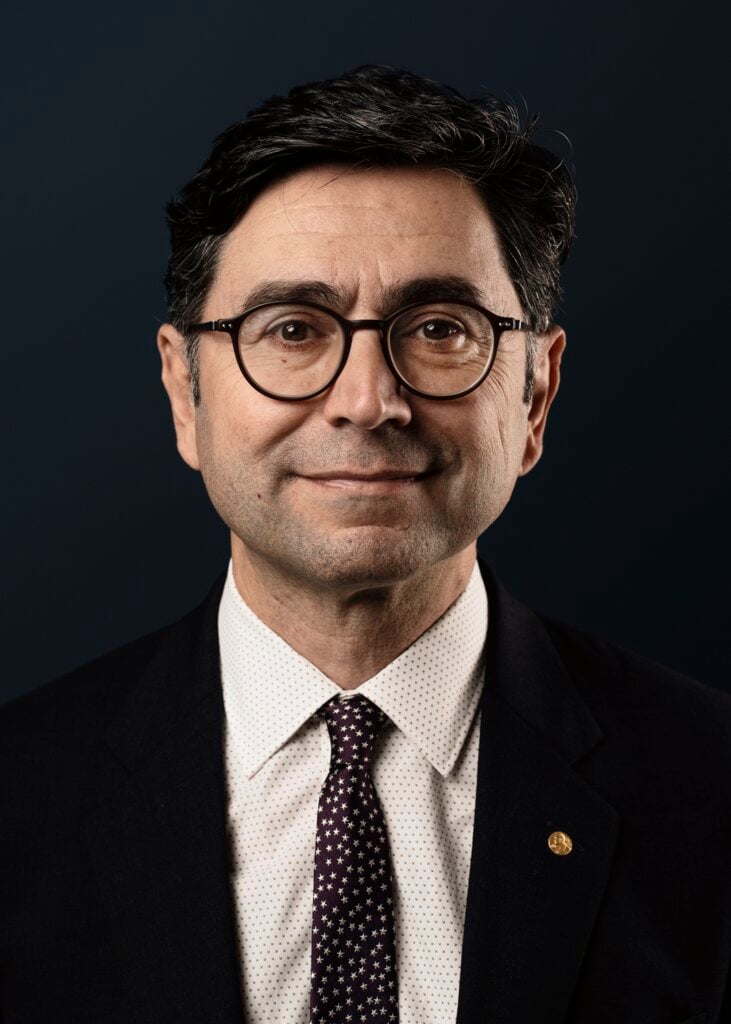
For the past two decades, I have studied the molecules that convey our sense of touch. I entered this field pursuing the allure of basic biology research, never anticipating the many directions these findings would take. Indeed, my life has been full of directions I never imagined. As an 18-year-old refugee from Lebanon, I had no idea I would become a scientist, and certainly not the recipient of a Nobel Prize.
From Lebanon to Los Angeles
I am of Armenian descent, born in Beirut, Lebanon, in 1967. My mother, Haigouhy Ajemian, is a retired elementary school teacher and principal, with a degree in biology; my father, Sarkis Patapoutian, is a retired accountant and a writer, under the pen name Sarkis Vahakn. My older brother, Ara, is an electrical engineer; my older sister, Houry, an architect and teacher. My early childhood was typical, at times even idyllic – I recall the beauty of Lebanon, the delicious cuisine, visits to the Mediterranean Sea and the surrounding mountains, and running wild in nature in the summer months.
But in 1975, clashes erupted between religious factions in Beirut. Armenians like my family were mostly thought of as neutral observers to the conflicts between Christians and Muslims, but times were tough for everyone. Beirut’s infrastructure – both physical and cultural – began to deteriorate, and lives were upended by curfews, limited hours of electricity, lack of running water, and bombings of the city. Still, between the blasts, I had many of the trappings of a usual childhood; I quite fondly remember playing basketball and table tennis, reading Tintin, and spending a lot of time watching TV.
I attended small private Armenian schools in Beirut, first Demirdjian and then Hovagimian-Manougian, both located not far from the Green Line that separated Muslim West Beirut from Christian East Beirut. Each year, though, my class grew smaller: Armenian families one by one moved away to escape the war. By my freshman year of high school, I only had four peers in my grade. I was ranked third out of the five of us, an average student. The school closed the next year.
I was a late bloomer, and it wasn’t until I enrolled at a new school, the more academically challenging Rawdah High School, that I began to discover how much I loved math and science classes. The bar was set high as I followed in Ara’s footsteps, and I still remember the first day of class in 10th grade when the physics teacher recognized my last name and asked if I was as scholarly as Ara. “I hope so,” I answered. After graduation, I enrolled at the American University of Beirut, a sprawling and lush campus overlooking the Mediterranean Sea. With my newfound interest in science, I declared a pre-med major; I really didn’t know, at the time, that being a scientist was a career option.
One frightening day, however, changed everything. I lived in West Beirut and had spent the night after a party at a friend’s house in East Beirut. The next morning while crossing the border between the halves of the city, I heard sniper shots. Terrified, I started running. As I sprinted into West Beirut, a group of militants motioned me toward them – a young man running across the Green Line seemed suspicious.
The militants held me for a day, at one point threatening to shoot me in the knee to see if I could feel pain. If I couldn’t feel pain, they said, it meant I was a spy. I responded, rather foolishly, “Couldn’t I just pretend to feel pain?” Eventually, the men realized I was harmless and let me go. But that was the final straw for me.
Despite the drawbacks of leaving the only life I knew behind, I began making plans to emigrate. A few months later, I packed my bags and flew to Los Angeles.
Discovering Science
Your body uses a sense called proprioception to help you stay afoot. Sensory cells innervating your muscles inform your brain of your precise posture and place in space. Without this sense, you’d struggle to stay upright and balanced. When you find yourself on rocky ground, proprioception is especially useful. My first months in the United States were, in a metaphorical sense, rocky. It wasn’t until years later, of course, that I learned about proprioception – a sense that, along with touch, my lab would help explain at the molecular level.
My goal was to continue my pre-med trajectory at the University of California, Los Angeles (UCLA). But first there were the common immigrant struggles to face – adapting to a new country, earning some money, and gaining admission to the school. To establish residency in California, I first spent a year working, delivering pizzas and writing horoscopes for an Armenian newspaper. Although I’d grown up watching English language television and taking English classes in school, I initially struggled to understand people speaking around me in Los Angeles. Slowly, as I figured out the pace and language of LA, I found my footing.
A year later, I was accepted to UCLA to study chemistry as a pre-med student, eventually changing my major to biochemistry and then biology. I knew very little about the revolution in molecular biology dominating research in the latter half of the twentieth century. Professor Bob Goldberg taught an introductory molecular biology class called Biology 7, where I first learned the power of DNA and genetics to describe human physiology and disease at a level unimaginable by prior generations. Dr. Goldberg also had us read The Double Helix by James D. Watson, who shared the 1962 Nobel Prize in Physiology or Medicine for co-discovering the structure of DNA. Today I feel Jim’s legacy is compromised, but his book portrayed the fun of basic science and had a huge impression on me.
Hoping to round out my pre-med curriculum and get a letter of recommendation for medical school, I was fortunate to join Professor Judy Lengyel’s research lab focused on the fruit fly Drosophila. It was there that I fell in love with research. It was the late 1980s, an exciting and rapidly changing time for fly genetics, and I learned molecular biology from Lengyel’s graduate students Eiríkur Steingrímsson and Richard Baldarelli. Although I was an inexperienced undergraduate, they let me join in their experiments as they hunted for the genes critical for fly development. The lab discovered tailless, the gene that establishes anterior and posterior polarity in Drosophila embryos. Our results on tailless were published in 1990, with me listed as the fifth author, one of my most treasured publications to this day.
Working in a lab felt completely different from anything else that I had done before. I loved the camaraderie, the flexible hours, and the drive to discover. Working as part of a team, with a diverse group of other scientists, was addictive. When I found out that graduate school–but not medical school – paid a monthly living stipend, I made up my mind. I dropped my dream of medical school (or was it my parents’ dream?) and applied to PhD programs in biology.
I was very happy to stay in LA for graduate school at California Institute of Technology (Caltech), where I continued studying transcriptional regulation, this time in the context of muscle differentiation. I joined the lab of Barbara Wold, a big thinker who would go on to co-found Caltech’s Gene Expression Center and direct the Beckman Institute at Caltech. She developed and adopted new technologies and taught us all to look at science broadly, rather than getting slowed down by the small details of a mature field. I embraced lab work and once again learned so much from senior students in the lab, including Jeff Miner and Paul Garrity, as well as from contemporaries such as Kyuson Yun.
I loved my years at Caltech, a small and beautiful campus full of curious folks who are very passionate about research. There was very little bureaucracy, and we had easy access to professors, often worldwide leaders in their fields. I worked long hours in the lab, often late into the night and over the weekend. But always a fan of work/life balance, I also fit in various hobbies: doing photography, running, and cooking at the Prufrock House, where I lived as a graduate student. By 1996, I had defended my thesis and published three papers with Wold that described my graduate work – a look at how cells decide to become muscle cells during development. Most of our work in the Wold lab at the time was focused on a small group of transcription factors, that when expressed in naïve cells, were sufficient to induce myogenesis. This concept that one gene can regulate a whole developmental program was very powerful and got me hooked for life on finding other genes that play a central role in physiology.
Also important during this time, I met my future wife, Nancy Hong, an undergraduate Biology student at Caltech. Over these past 32 years, we have grown up together, discussing science in the car and at the dinner table, tackling the joys and challenges of our science-related careers. I am a far better scientist today thanks to her input on my papers, grants, and talks.
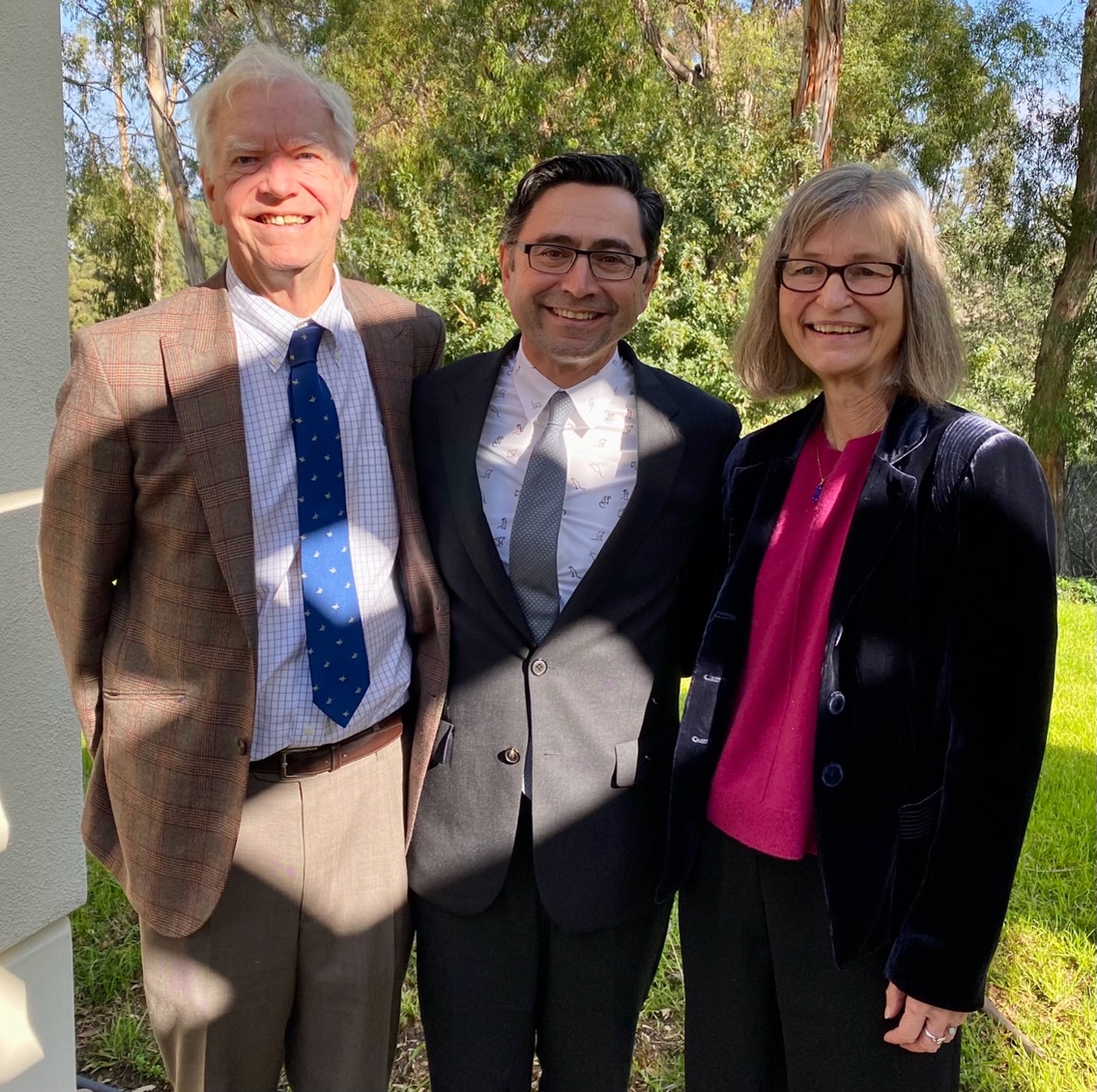
A New Interest in Sensory Neurons
After graduating from Caltech, I joined the lab of Louis Reichardt at the University of California, San Francisco (UCSF), for postdoctoral work from 1996 to 2000. Lou is one of a kind. An accomplished scientist, he was one of the founding editors of the journal Neuron, a Howard Hughes Medical Institute (HHMI) Investigator, and head of the neuroscience graduate program at UCSF. However, in some circles, he was more famous for being a world-class mountaineer. He was a member of the first American expedition to climb K2, the second highest peak on Earth. He also climbed Mt. Everest using a route never before attempted. As Isabel Farinas, a senior postdoctoral fellow, said to me when I joined the lab, “Don’t ever tell Lou something can’t be done.” Lou became my role model for how to run a lab, giving his students and fellows a great deal of independence at the same time as being a strong supporter. He almost never stopped by to check on work progress but was always available to chat when you wanted or needed it.
As in previous labs, my fellow researchers also became mentors and good friends. Uli Mueller, a senior postdoc, seemed to spend a good part of his last year in Lou’s lab distracting me and another junior postdoc, Ralph Brandenberger, with endless invitations to coffee. We enjoyed great discussions during these coffee breaks, gossiping about science and scientists and fending off Uli’s challenges to the importance of our projects. I continued to learn how to focus on questions that I could defend as truly impactful if answerable.
In Lou’s lab, I turned to studying the somatosensory neurons that initiate touch and pain. Lou let me start a variety of projects, many of which did not develop into full-fledged efforts. But three eventually grew into related insights on how signaling proteins called neurotrophins drive the survival and specialization of sensory neurons.
After five years in lovely San Francisco, I decided to embark on my independent research career at an unconventional new enterprise led by Pete Schultz. Renowned as one of the top chemists in the world, Pete is also an entrepreneur who has founded many biotech companies. He was leaving his UC Berkeley and HHMI positions to join Scripps Research in San Diego and to create a genomics institute funded by Novartis Research Foundation, a nonprofit associated with the Swiss drug company. I began a joint position with these two institutions in 2000, just as the sequence of the human genome was being completed. The opportunity to use novel genomics technologies to address fundamental questions in biology was extremely exciting.
I established my own lab and continued studying the development of somatosensory neurons: how does a temperature-sensitive neuron decide to become a so-called “thermosensor” while neighboring neurons become specialized in sensing touch? As interesting as this developmental biology question was, I also realized that another fundamental question about the function of these cells remained a huge mystery. Instead of sensing chemicals (e.g., hormones or neurotransmitters), these neurons sense physical stimuli such as temperature and pressure. How they accomplish this at the molecular level was mostly unknown. I felt we could use genomics tools to identify the molecular receptors for temperature and pressure.
Somatosensory neurons like those that I studied with Lou stretch from the tips of a person’s extremities to the spinal cord and all the way to the brain, carrying messages about temperature, touch, itch, and pain. At their ends, ion channels receive the initial messages about these senses. The channels are tiny – a few nanometers in diameter – but vital to cells’ functioning. In response to stimuli, ion channels open and close, changing the flow of ions in and out of cells and thus changing the voltage of the cells’ membranes, the language of the nervous system.
The electrophysiological properties of temperature and mechanically activated ion channels had been studied, but their molecular identities remained unknown. Back at UCSF, David Julius had just demonstrated TRPV1 to be the first ion channel activated by heat. Armed with early sequence data from the human genome, we asked if other TRP ion channels were involved in thermosensation. In a few years, we identified a channel activated by both cold temperatures and menthol, the cooling compound derived from mint leaves. We published our results in 2002, dubbing the channel TRPM8, at the same time David’s lab published very similar independent findings.
Over the next couple years, my young lab further characterized TRPM8 and identified other somatosensory ion channels, including TRPV3 and TRPA1. TRPA1 can be activated by noxious cold and plays a role in sensing reactive chemicals to transduce a painful signal. Indeed, we and others have shown that the burning of mustard oil (wasabi), wintergreen oil (a key ingredient in mouthwashes such as LISTERINE®), and raw garlic all act through TRPA1. This link between TRPA1 and pain led several pharmaceutical companies to research TRPA1 blockers for various types of clinical pain in humans. It was an important lesson that studying the basic biology of sensory ion channels could have further consequences for medicine.
The Sense of Touch
After working for a decade on temperature-activated sensory channels, I felt that the field was maturing. Many scientists were focused on TRP ion channels now, and I thought it was time to ask a new question: Which channels sense mechanical forces, allowing touch and pain? Hearing, sensing blood pressure, and many other critical biological processes depend on mechanically activated ion channels, and yet the molecules responsible for detecting pressure were mainly unknown.
What was the best way to identify these unknown channels? We quickly shelved efforts to identify them directly from the somatosensory neurons responsible for touch. Working with these finicky and heterogenous cells in the lab was difficult enough; trying to isolate channels from them seemed nearly impossible. Instead, postdoctoral fellow Bertrand Coste spearheaded a new assay to determine cell types that would respond to pressure and could be easily and homogeneously grown in culture. We reasoned that this might lead us toward a pressure-sensing channel that, while discovered elsewhere, could turn out to be relevant in sensory cells.
For this purpose, Bertrand screened cell lines, recording the electrical activity of a cell while poking it with a glass probe. If the cell expressed a mechanically activated channel, we expected to see changes to the current when it was pushed. He found that Neuro2A cells were exquisitely mechanosensitive and decided to focus on these cells.
Next, Bertrand worked through a list of 300 genes expressed in Neuro2A cells that might possibly encode an ion channel. He knocked each gene down using RNA interference (RNAi) molecules, and then once again recorded the effect of poking the cell on its electrical current. For a year, no gene seemed to have an effect on the ability of the Neuro2A cells to sense pressure. But on the 72nd candidate that Bertrand tested, removing the gene wiped out the currents. I recall that – despite the “Eureka!” potential for the moment – Bertrand came to my office very calmly and told me, “I found it.” He knew the importance of what he had discovered.
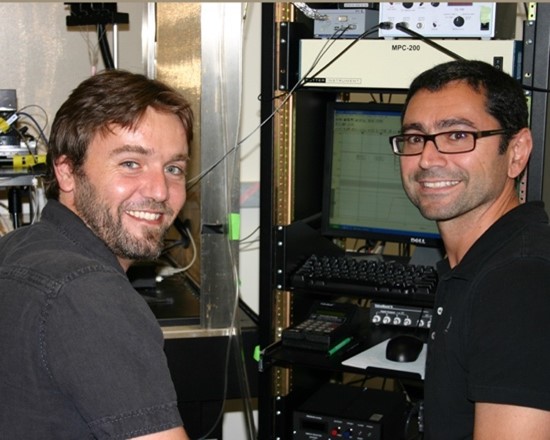
We named the new protein PIEZO1, after the Greek word for pressure, piezi. In 2010, we reported the results: not only did removing PIEZO1 from Neuro2A cells inhibit their ability to sense pressure, but adding PIEZO1 to embryonic kidney cells gave them the new ability to respond to mechanical force. PIEZO1 is necessary and sufficient for producing mechanically activated currents, a double condition cherished by biologists. Our reductionist approach to finding a molecule responsible for mechanically activated currents in Neuro2A cells and hoping to extend that to touch sensation was quite a leap. I am glad that Bertrand took that leap with me!
Uncovering Piezo Biology
When we searched the genome for other genes related to PIEZO1, we quickly turned up another protein, which we named PIEZO2. In the years after our discoveries of PIEZO1 and PIEZO2, we undertook a range of projects to more fully characterize the biology of these two ion channels – determining their structures, which cells they were expressed in, and what biological functions they play roles in.
An important milestone was showing PIEZO2 is robustly expressed in sensory neurons. This was followed by experiments demonstrating that PIEZO2 is the principal in vivo sensor of light touch, as mice without PIEZO2 are not able to sense vibrations or detect a piece of tape attached to their back. Importantly, we also showed that tactile allodynia (a condition in which touch becomes painful, such as after a sunburn), is also dependent on PIEZO2, raising the possibility that PIEZO2 could be a relevant target for neuropathic pain. But as my colleagues and I continued analyzing the effects of PIEZO mutations, we found that the channels go far beyond allowing us to sense a gentle breeze or a pinch on the arm. PIEZO2 also helps control other important functions by giving the body feedback on the pressure and stretch experienced by internal organs. PIEZOs sense how much a person’s lungs stretch with each breath – information that’s used to control breathing rate. They sense the pressure exerted by blood moving through the aortic arch, which then mediates blood pressure changes. They also tell us when the bladder is stretched out and full.
I also became especially interested in the concept of proprioception – that sense of where your body is in space. I think in many ways it is the most important of our senses, even though most people have never heard of it. We found that, indeed, mice without the PIEZO2 ion channel lack coordination. In a fascinating turn of events, Alex Chesler and his collaborators at the NIH, identified and characterized human subjects who are born without PIEZO2. Remarkably, many of the deficits described for mutant PIEZO2 mice are also experienced by humans without PIEZO2. For example, PIEZO2-deficient individuals don’t sense touch, struggle to learn to walk due to lack of proprioception, and don’t have a sense of their bladder being full. All this work on somatosensation and interoception was carried out by a dedicated group of graduate students and postdocs in my lab as well as collaborators across the globe. While there are too many important contributors to be mentioned here by name, I am forever indebted to them.
Pressure Sensing Beyond the Nervous System
One of the most rewarding features of science is the unanticipated direction it may lead you. Most recently, my team has launched projects looking at the role of PIEZO1 in non-neuronal cells. Scientists know that many different cell types experience, sense, and respond to mechanical forces. But exactly how they sense forces – and in many cases, why – is unknown. My lab and others have in recent years found important roles of PIEZO1 in the development of the cardiovascular system, bone formation, and in red blood cells, where it seems to have an interesting connection to malaria. Distant relatives of PIEZO can be found in plants and unicellular organisms. Indeed, we recently showed that PIEZO is expressed in the tips of plant roots, and without it, the roots can’t penetrate hard surfaces or soil. From proprioception to malaria to plant roots, research that began with a simple, fundamental question in sensory neurobiology has already taken me on a remarkable journey, and I intend to keep at it for years to come.
Looking Back
When the Nobel Prize call was made – at two in the morning California time – my phone was set to “do not disturb.” But the committee was able to reach my 94-year-old father, who was then the one to give me the news.
Amidst the celebrations with my family, friends, colleagues and collaborators, the Nobel Prize also helped me reconnect with my roots. I am the first Nobel laureate of Armenian origin as well as the first from Lebanon, so both communities celebrated from afar.
When I reflect on my career, I credit my mentors, collaborators, and trainees for much of my success. My time in the Wold and Reichardt labs imparted on me not only my love of science, but examples of how to run collegial, high-achieving, fun lab groups, and I have strived to do the same ever since. I have emphasized the importance to collaborate. Bringing researchers together who have different expertise and backgrounds creates a rich, fun environment and is a more effective way to do science.
I also learned during my years as a trainee that science is very hard, and that if your projects are ambitious, many if not most will fail. As Lou told me a while back, batting 1 out of 3 in baseball is considered a great feat. Good ideas can come from well-researched experimental plans, but they can also come from something as vague as an informed intuition. So it is crucial at times to trust your training and knowledge, and to take a leap as Bertrand did. In addition, although it is important to have bold ideas, it is equally imperative to know when to let go of the ones that aren’t working out.
Lastly, securing funding and publishing results are two of the biggest frustrations researchers face. For example, our first grant application proposing to find out whether PIEZO2 was the touch/pain sensor was rated in the bottom half of applications and rejected. And the manuscript showing definitive evidence that PIEZO2 is the touch sensor was declined by two journals before finding its home. I mention this to remind young investigators that they are not alone facing such difficulties and to persevere in the face of negative feedback. In my experience, well-supported science will eventually prevail.
Having learned all these lessons, I have advised my trainees to initiate multiple projects early on, and I work with them to monitor progress through a reiterative process of prioritizing and editing. To watch my students and postdocs go on to establish themselves as independent scientists in academia and industry is one of my great pleasures.
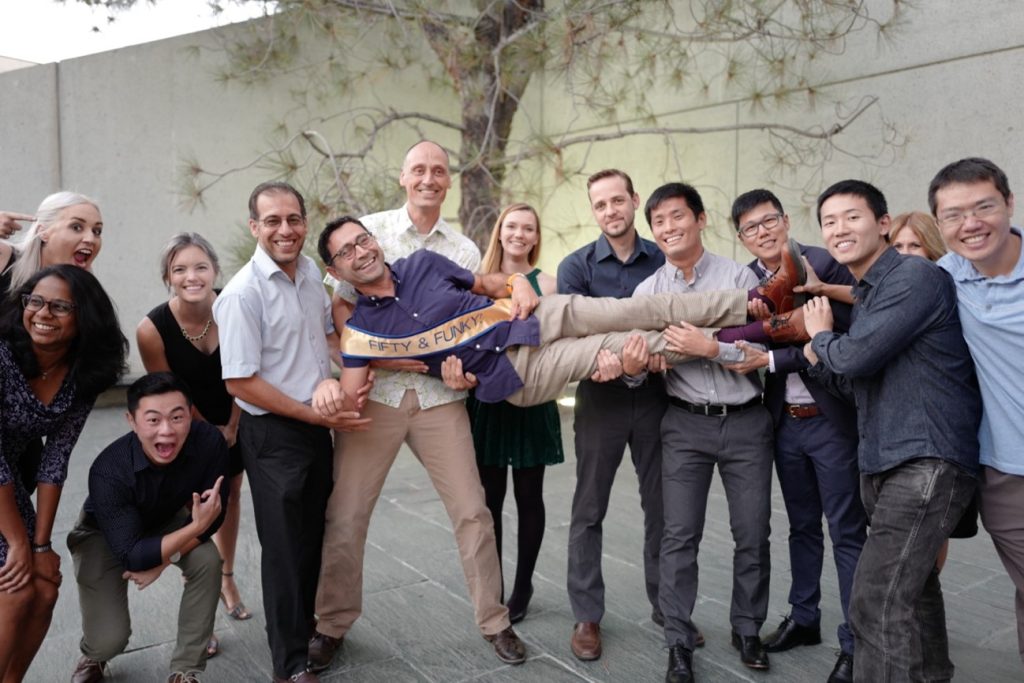
Individual laboratories do not function by themselves. The institutions I have belonged to have also been critical, allowing me to explore my interests and let my results take me in new directions. Indeed, I so enjoy my wonderful faculty colleagues at Scripps Research who not only enrich my science but also join me for swims in the Pacific Ocean and backpacking in the Sierra Nevada mountains. Biomedical research is expensive, and I am thankful for funding from the National Institutes of Health and the Howard Hughes Medical Institute.
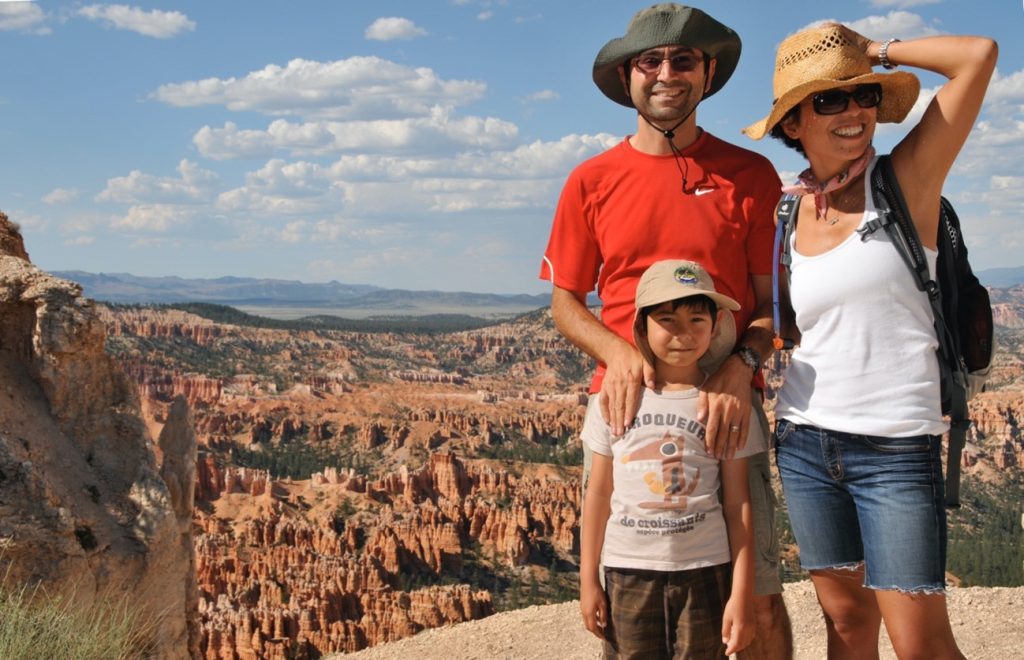
As mentioned, I am a big proponent of work/life balance, and I have always prioritized my family over work. Nancy has been my full partner in this journey, and our son, Luca, has been such a wonder to watch grow. Through my uncertain times as a youth, my parents and siblings always prioritized education and unwavering love.
Finally, I am immensely privileged to be a scientist. I feel such joy to be on this incredible intellectual journey, to be working with diverse and dedicated colleagues, and to be experiencing amazing opportunities that science has given me, all while unraveling the mysteries of biology. Prizes are brilliant tools to recognize science that impacts humanity and to hopefully inspire the next generation; however, they work less well as the goal of a scientist, at least in my opinion. The Nobel Prize, in particular, brings a great deal of attention to scientists. This can be dizzying. As I adjust to this new recognition, I remind myself that the Nobel Prize can be granted to ordinary people who have been lucky enough to discover extraordinary results.
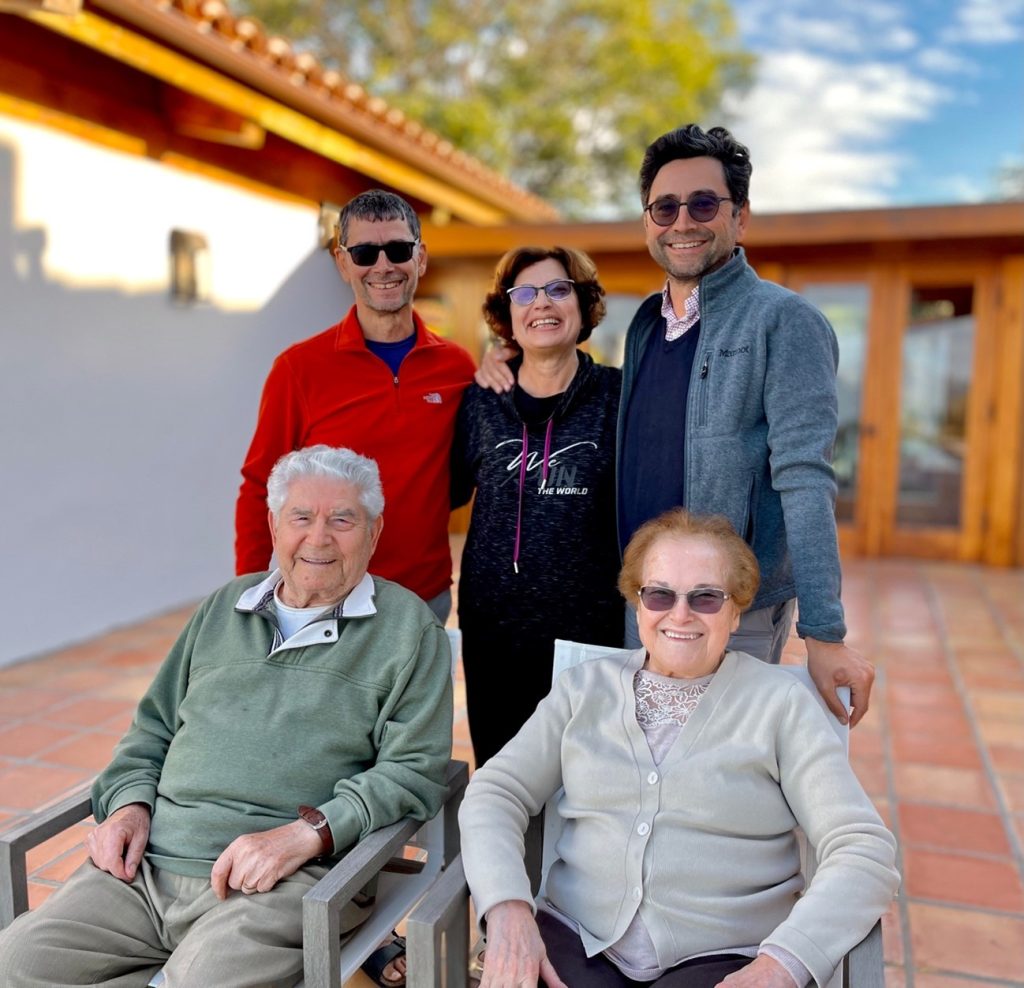
© The Nobel Foundation 2023
Nobel Prizes and laureates
See them all presented here.