Popular information
Popular science background:
Taking the experiment to cyberspace (pdf)
Populärvetenskaplig information:
De flyttade experimenten till cyberrymden (pdf)
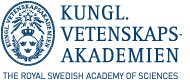
The Nobel Prize in Chemistry 2013
Chemical reactions occur at lightning speed; electrons jump between atoms hidden from the prying eyes of scientists. The Nobel Laureates in Chemistry 2013 have made it possible to map the mysterious ways of chemistry by using computers. Detailed knowledge of chemical processes makes it possible to optimize catalysts, drugs and solar cells.
Taking the experiment to cyberspace
Chemists all over the world devise and carry out experiments on their computers on a daily basis. With the help of the methods that Martin Karplus, Michael Levitt and Arieh Warshel began to develop in the 1970s, they examined every tiny little step in complex chemical processes that are invisible to the naked eye.
In order for you, the reader, to get an idea of how mankind can benefit from this, we begin with an example. Put your lab coat on, because we have a challenge for you: to create artificial photosynthesis. The chemical reaction occurring in green leaves fills the atmosphere with oxygen and is one prerequisite for life on Earth. But it is also interesting from an environmental perspective. If you can mimic the photosynthesis you will be able create more efficient solar cells. When water molecules are split oxygen is created, but also hydrogen that could be used to power our vehicles. So there is ample reason for you to get engaged in this project. If you succeed, you could contribute to solving the problem with greenhouse effect.
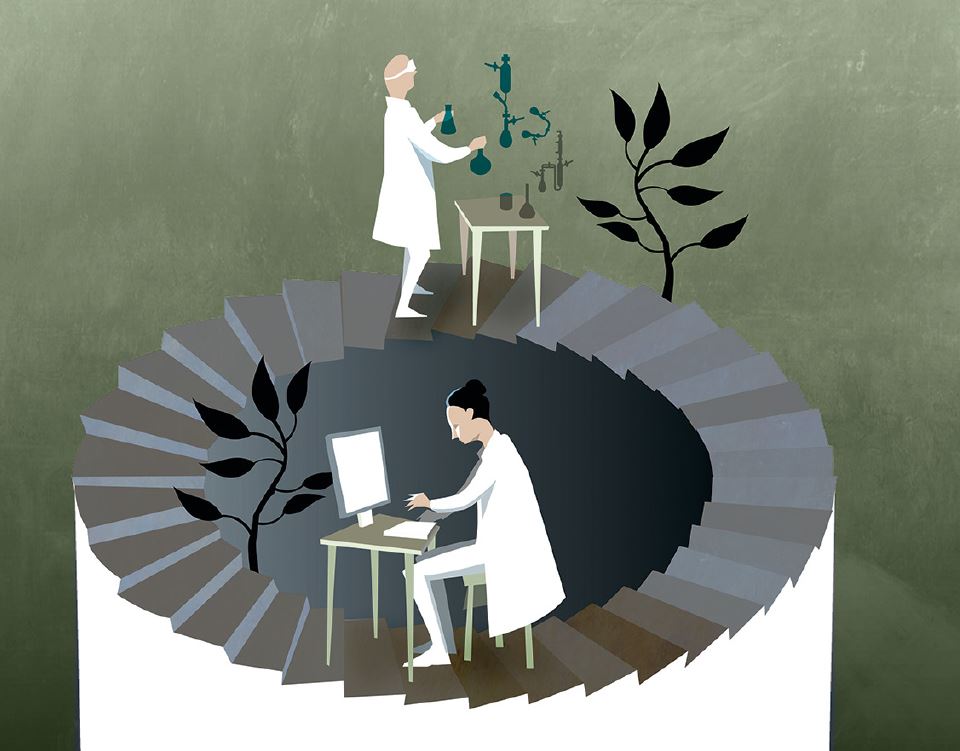
A picture says more than a thousand words – but not everything
As a first step you will probably go online and find a three dimensional image of the proteins that govern the photosynthesis. Such images are freely accessible in large databases on the internet. In your computer you can twist and turn the image as you like. It unveils gigantic protein molecules consisting of tens of thousands of atoms. Somewhere in the middle, there is a little region called the reaction centre. This is where the water molecules are split. However, only a few atoms are directly involved in the reaction. Among other things, you see four manganese ions, one calcium ion and several oxygen atoms. The image clearly shows how atoms and ions are positioned in relation to each other, but it says nothing about what these atoms and ions do. This is what you need to find out. Somehow, electrons must be extracted from the water and four protons need to be taken care of. How does that happen?
The details of this process are virtually impossible to map using traditional methods of chemistry. Many things happen in a fraction of a millisecond – a rate that rules out most kinds of test tube experiments. From the image that you have in your computer, it is also difficult to guess the reaction process, because it was taken when the proteins were in a state of rest. When sunlight hits the green leaves, however, the proteins are filled with energy and the entire atomic structure is changed. In order to understand the chemical reaction you need to know what this energy-filled state looks like.
This is where you summon the help of the computer programs that the Nobel Laureates in Chemistry 2013 have laid the foundation for.
Theory and practice – a successful cross-fertilization
Using this kind of software you can calculate various plausible reaction pathways. This is called simulation or modelling. In this manner you can get an idea of what role specific atoms play at different stages of the chemical reaction. And when you have a plausible reaction path it is easier to carry out real experiments that can confirm whether the computer is right or not. These experiments, in turn, can yield new clues that lead to even better simulations; theory and practice cross-fertilize each other. As a consequence, chemists now spend as much time in front of their computers as they do among test tubes.
So what is so special about the computer programs that now are awarded with the Nobel Prize in Chemistry?
Combining the best of both worlds
Previously when scientists wanted to simulate molecules on computers, they had software at their disposal that was based upon either classical Newtonian physical theories or quantum physics. Both had their strengths and weaknesses. The classical programs could calculate and process large chemical molecules. They would only display molecules in a state of rest, but gave chemists a good representation of how the atoms were positioned in the molecules. However, you could not use these programs to simulate chemical reactions. During the reaction, the molecules are filled with energy; they become excited. Classical physics simply have no understanding for such states, and that is a severe limitation.
When scientists wanted to simulate chemical reactions, they had to turn to quantum physics; the dualistic theory where electrons can be both particles and waves simultaneously and where Schrödinger’s famous cat, hidden in its box, can be both alive and dead. The strength of quantum physics is that it is unbiased and the model will not include any of the scientist’s preconceptions. Therefore such simulations are more realistic. The downside is that these calculations require enormous computing power. The computer has to process every single electron and every atomic nucleus in the molecule. This can be compared to the number of pixels in a digital image. Many pixels will give you a high resolution, but also require more computer resources. Similarly, quantum physical calculations yield detailed descriptions of chemical processes, but require powerful computers. In the 1970s, this meant that scientists could only perform calculations on small molecules. When modelling, they were also forced to ignore interactions with the surrounding environment, although chemical reactions in real life most often occur in some kind of solution. However, if scientists would have wanted the computer to include the solvent in the calculation, they would have had to wait decades for the results.
So, classical and quantum chemistry were two fundamentally different, and in some respects rivalling, worlds. But the Nobel Laureates in Chemistry 2013 have opened a gate between these two worlds. In their computer models, Newton and his apple collaborate with Schrödinger and his cat.
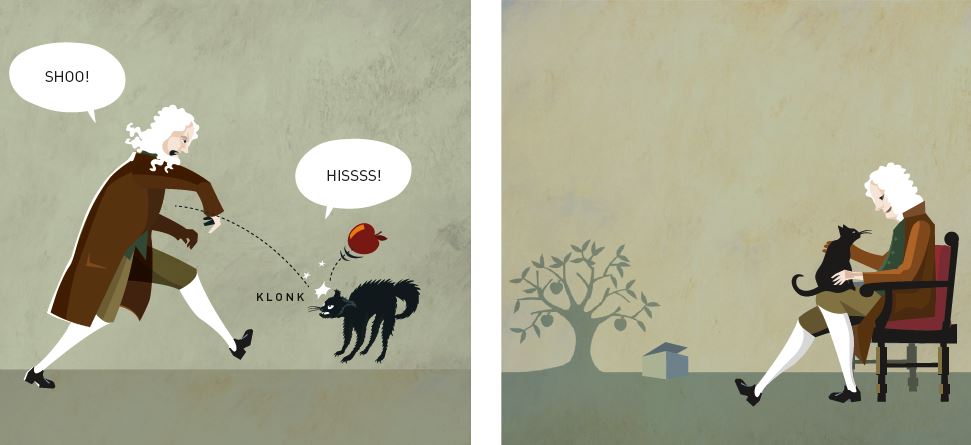
Quantum chemistry collaborating with classical physics
The first step towards this collaboration was taken at the beginning of the 1970s in Martin Karplus’ laboratory at Harvard University in Cambridge, USA. Karplus was firmly rooted in the quantum world. His research group developed computer programs that could simulate chemical reactions with the help of quantum physics. He had also developed the “Karplus equation”, which is used in nuclear magnetic resonance (NMR); a method well-known to chemists that builds on the quantum chemical properties of molecules. Upon finishing his PhD, Arieh Warshel arrived at Karplus’ laboratory in 1970. He had received his doctoral training at the Weizmann Institute of Science in Rehovot, Israel. The institute had a powerful computer, the Golem, named after a creature in Jewish folklore. With the help of Golem, Arieh Warshel and Michael Levitt had developed a ground-breaking computer program based on classical theories. The program enabled modelling of all kinds of molecules, even really large biological molecules.
When Arieh Warshel joined Martin Karplus at Harvard, he brought his classical computer program with him. Using that as a point of departure, he and Karplus began developing a new kind of program that performed different kinds of calculations on different electrons. In most molecules each electron orbits a particular atomic nucleus. But in some molecules, certain electrons can move unhindered between several atomic nuclei. Such “free electrons” can be found, for instance, in retinal, a molecule embedded in the retina of the eye. Karplus had a longstanding interest in retinal as the quantum chemical properties of the molecule affected a certain biological function; when light hits the retina, the free electrons in retinal are filled with energy, which alters the shape of the molecule. This is the first stage of human vision.
Eventually Karplus and Warshel managed to model retinal. However, they started out with similar molecules of a more simple structure. They developed a computer program that drew on quantum physics when it performed calculations on free electrons, and applied more simple classical theories for all other electrons and all atomic nuclei. In 1972, they published their results. This was the first time anyone had managed to bring about a chemically relevant collaboration between classical and quantum physics. The program was ground-breaking but had one limitation. It could only handle molecules with mirror symmetry.
A universal program for calculating the chemistry of life
After two years at Harvard, Arieh Warshel reunited with Michael Levitt. Levitt had now finished his doctoral training at Cambridge University, UK; at the time world-leading in the study of biological molecules such as DNA, RNA and proteins. He had used his classical computer program in order to gain a better understanding of what biological molecules looked like. The limitation remained, however; it was only possible to examine molecules in a state of rest.
Levitt and Warshel aimed high. They wanted to develop a program that could be used to study enzymes; proteins that govern and simplify chemical reactions in living organisms. Already as a young student, Warshel had become curious about how enzymes function. It is the cooperation between enzymes that makes life possible. They control virtually all chemistry in the living body. If you want to understand life, you need to understand enzymes.
In order to be able to simulate enzymatic reactions, Levitt and Warshel were required to make classical and quantum physics collaborate more smoothly. It would take them several years to overcome all obstacles. They began their explorations at the Weizmann institute in Rehovot, but when Levitt a few years later finished his post-doctoral training, he moved back to Cambridge where he was joined by Warshel. In 1976, they reached their goal and published the first computerized model of an enzymatic reaction. Their program was revolutionary because it could be used for any kind of molecule. Size was no longer an issue when simulating chemical reactions.
Focusing on the heart of the action
When chemists model chemical processes today, they apply the power where it is needed. They perform demanding quantum physical calculations on the very electrons and atomic nuclei that directly impact the chemical process. In that way, they get the best possible resolution where it matters. The other parts of the molecules are modelled using classical equations.
In order not to waste computing power, Michael Levitt and Arieh Warshel have trimmed the calculation workload even further. The computer does not always have to account for every single atom in the less interesting parts of the molecule. They have showed that it is possible to merge several atoms during the calculations.
In modern calculations, scientists also add a third layer to the simulation. Put in a somewhat simplified manner, the computer can, for areas very far away from the chemical process, bundle atoms and molecules into a single homogenous mass. In the scientific community this is referred to as dielectric medium.
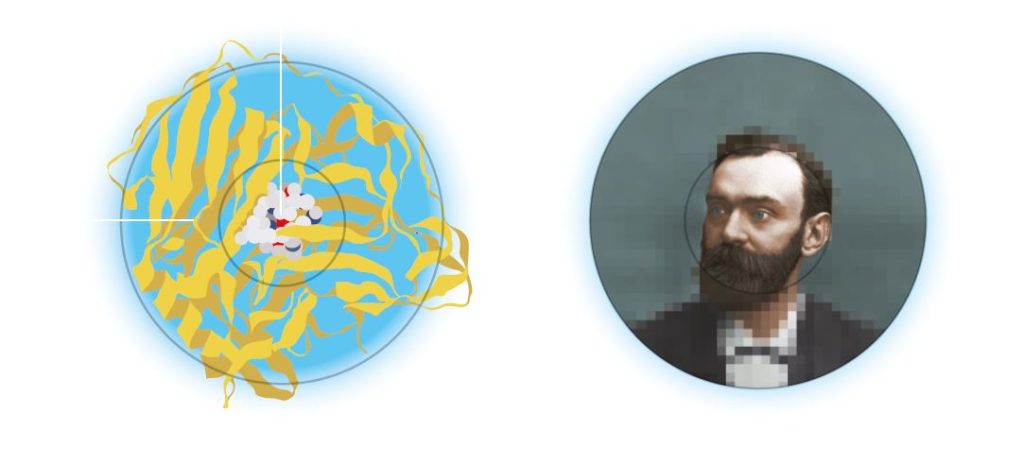
How far the simulations will take us is for the future to decide
The fact that scientists these days can use computers to carry out experiments has yielded a much deeper understanding of how chemical processes play out. The strength of the methods that Martin Karplus, Michael Levitt and Arieh Warshel have developed is that they are universal. They can be used to study all kinds of chemistry; from the molecules of life to industrial chemical processes. Scientists can optimize solar cells, catalysts in motor vehicles or even drugs, to take but a few examples.
Progress will not stop there, however. In one of his publications, Michael Levitt writes about one of his dreams: to simulate a living organism on a molecular level. It is a tantalizing thought. The computer models that have been developed by the Nobel Laureates in Chemistry 2013 are powerful tools. Exactly how far they can advance our knowledge is for the future to decide.
Links and further reading
Articles
Levitt, M. (2001) The birth of computational structural biology, Nature structural biology 8:392–393.
Karplus, M. (2006) Spinach on the Ceiling: A Theoretical Chemist’s Return to Biology, Annu. Rev. Biophys. Biomol. Struct. 35: 1–47.
Johnson, P. (2012) Warshel Fêted by Royal Society of Chemistry, http://128.125.126.117/news/stories/1298/
warshel-fted-by-royal-society-of-chemistry/
The Laureates
MARTIN KARPLUS
U.S. and Austrian citizen. Born 1930 in Vienna, Austria. Ph.D. 1953 from California Institute of Technology, CA, USA. Professeur Conventionné, Université de Strasbourg, France and Theodore William Richards Professor of Chemistry, Emeritus, Harvard University, Cambridge, MA, USA
http://chemistry.harvard.edu/people/martin-karplus
http://www-isis.u-strasbg.fr/biop/start
MICHAEL LEVITT
U.S., British and Israeli citizen. Born 1947 in Pretoria, South Africa. Ph.D. 1971 from University of Cambridge, UK. Robert W. and Vivian K. Cahill Professor in Cancer Research, Stanford University School of Medicine, Stanford, CA, USA.
http://med.stanford.edu/profiles/Michael_Levitt
ARIEH WARSHEL
U.S. and Israeli citizen. Born 1940 in Kibbutz Sde-Nahum, Israel. Ph.D. 1969 from Weizmann Institute of Science, Rehovot, Israel. Distinguished Professor, University of Southern California, Los Angeles, CA, USA.
http://med.stanford.edu/profiles/Michael_Levitt
Science Editors: Sven Lidin, Gunnar Karlström, the Nobel Committee for Chemistry
Text: Ann Fernholm
Illustrations: © Johan Jarnestad/The Royal Swedish Academy of Sciences
Editor: Perina Stjernlöf
© The Royal Swedish Academy of Sciences
Nobel Prizes and laureates
Six prizes were awarded for achievements that have conferred the greatest benefit to humankind. The 12 laureates' work and discoveries range from proteins' structures and machine learning to fighting for a world free of nuclear weapons.
See them all presented here.