Popular information
Popular science background:
Their tools revolutionised the construction of molecules (pdf)
Populärvetenskaplig information:
Deras verktyg har revolutionerat bygget av molekyler (pdf)
The Nobel Prize in Chemistry 2021
Chemists can create new molecules by linking together small chemical building blocks, but controlling invisible substances so they bond in the desired way is difficult. Benjamin List and David MacMillan are awarded the Nobel Prize in Chemistry 2021 for their development of a new and ingenious tool for molecule building: organocatalysis. Its uses include research into new pharmaceuticals and it has also helped make chemistry greener.
Their tools revolutionised the construction of molecules
Many industries and fields of research depend on chemists’ ability to build new and functional molecules. These could be anything from substances that capture light in solar cells or store energy in batteries, to molecules that can make lightweight running shoes or inhibit the progress of disease in the body.
However, if we compare nature’s ability to build chemical creations with our own, we were long stuck in the Stone Age. Evolution has produced incredibly specific tools, enzymes, for constructing the molecular complexes that give life its shapes, colours and functions. Initially, when chemists isolated these chemical masterpieces, they just looked at them in admiration. The hammers and chisels in their own toolboxes for molecular construction were blunt and unreliable, so they often ended up with lots of unwanted byproducts when they copied nature’s products.
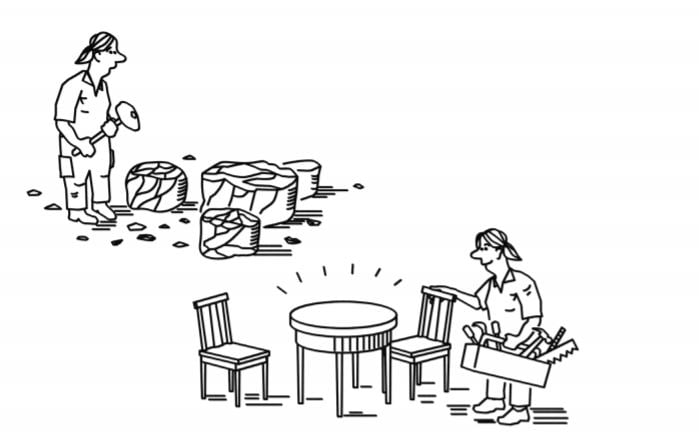
New tools for finer chemistry
Each new tool that chemists have added to their toolbox has increased the precision of their molecular constructions. Slowly but surely, chemistry has progressed from chiselling in stone to something more like fine craftsmanship. This has been of great benefit to humanity and several of these tools have been rewarded with the Nobel Prize in Chemistry.
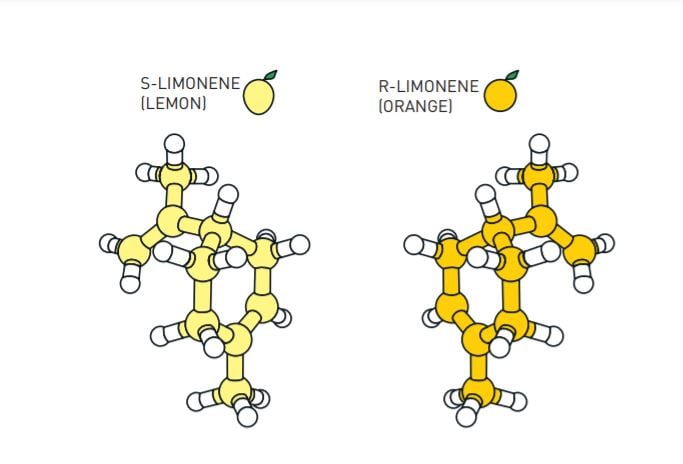
Many molecules exist in two variants, where one is the mirror image of the other. These often have completely different effects in the body. For example, one version of the limonene molecule has a lemon scent, while its mirror image smells like orange.
The discovery being awarded the Nobel Prize in Chemistry 2021 has taken molecular construction to an entirely new level. It has not only made chemistry greener, but also made it much easier to produce asymmetric molecules. During chemical construction a situation often arises in which two molecules can form, which – just like our hands – are each other’s mirror image. Chemists often just want one of these mirror images, particularly when producing pharmaceuticals, but it has been difficult to find efficient methods for doing this. The concept developed by Benjamin List and David MacMillan – asymmetric organocatalysis – is as simple as it is brilliant. The fact is that many people have wondered why we didn’t think of it earlier.
Why indeed? This is no easy question to answer, but before we even try we need to take a quick look back at history. We will define the terms catalysis and catalyst, and set the stage for the Nobel Prize in Chemistry 2021.
Catalysts accelerate chemical reactions
In the nineteenth century, when chemists began exploring the ways that different chemicals react with each other, they made some strange discoveries. For example, if they put silver in a beaker with hydrogen peroxide (H2O2), the hydrogen peroxide suddenly began to break down into water (H2O) and oxygen (O2). But the silver – which started the process – did not seem affected by the reaction at all. Similarly, a substance obtained from sprouting grains could break down starch into glucose.
In 1835, the renowned Swedish chemist Jacob Berzelius started to see a pattern in this. In the Royal Swedish Academy of Sciences’ annual report, describing the latest progress in physics and chemistry, he writes about a new “force” that can “generate chemical activity”. He listed several examples in which just the presence of a substance started a chemical reaction, stating how this phenomenon appeared to be considerably more common than was previously thought. He believed that the substance had a catalytic force and called the phenomenon itself catalysis.
Catalysts produce plastic, perfume and flavoursome food
A great deal of water has run through chemists’ pipettes since Berzelius’ time. They have discovered a multitude of catalysts that can break down molecules or join them together. Thanks to these, they can now carve out the thousands of different substances we use in our everyday lives, such as pharmaceuticals, plastics, perfumes and food flavourings. The fact is, it is estimated that 35 per cent of the world’s total GDP in some way involves chemical catalysis.
In principle, all catalysts discovered before the year 2000 belonged to one of two groups: they were either metals or enzymes. Metals are often excellent catalysts because they have a special ability to temporarily accommodate electrons or to provide them to other molecules during a chemical process. This helps loosen the bonds between the atoms in a molecule, so bonds that are otherwise strong can be broken and new ones can form.
However, one problem with some metal catalysts is that they are very sensitive to oxygen and water so, for these to work, they need an environment free of oxygen and moisture. This is difficult to achieve in large-scale industries. Also, many metal catalysts are heavy metals, which can be harmful to the environment.
Life’s catalysts work with astounding precision
The second form of catalyst is comprised of the proteins known as enzymes. All living things have thousands of different enzymes that drive the chemical reactions necessary for life. Many enzymes are specialists in asymmetric catalysis and, in principle, always form one mirror image out of the two that are possible. They also work side by side; when one enzyme is finished with a reaction, another one takes over. In this way, they can build complicated molecules with amazing precision, such as cholesterol, chlorophyll or the toxin called strychnine, which is one of the most complex molecules we know of (we will return to this).
Because enzymes are such efficient catalysts, researchers in the 1990s tried to develop new enzyme variants to drive the chemical reactions needed by humanity. One research group working on this was based at the Scripps Research Institute in southern California and was led by the late Carlos F. Barbas III. Benjamin List had a postdoctoral position in Barbas’ research group when the brilliant idea that led to one of the discoveries behind this year’s Nobel Prize in Chemistry was born.
Benjamin List thinks outside the box…
Benjamin List worked with catalytic antibodies. Normally, antibodies attach to foreign viruses or bacteria in our bodies, but the researchers at Scripps redesigned them so they could drive chemical reactions instead.
During his work with catalytic antibodies, Benjamin List started to think about how enzymes actually work. They are usually huge molecules that are built from hundreds of amino acids. In addition to these amino acids, a significant proportion of enzymes also have metals that help drive chemical processes. But – and this is the point – many enzymes catalyse chemical reactions without the help of metals. Instead, the reactions are driven by one or a few individual amino acids in the enzyme. Benjamin List’s out-of-the-box question was: do amino acids have to be part of an enzyme in order to catalyse a chemical reaction? Or could a single amino acid, or other similar simple molecules, do the same job?
…with a revolutionary result
He knew that there was research from the early 1970s where an amino acid called proline had been used as a catalyst – but that was more than 25 years ago. Surely, if proline really had been an effective catalyst, someone would have continued working on it?
This is more or less what Benjamin List thought; he assumed that the reason why no one had continued studying the phenomenon was that it had not worked particularly well. Without any real expectations, he tested whether proline could catalyse an aldol reaction, in which carbon atoms from two different molecules are bonded together. It was a simple attempt that, amazingly, worked straight away.
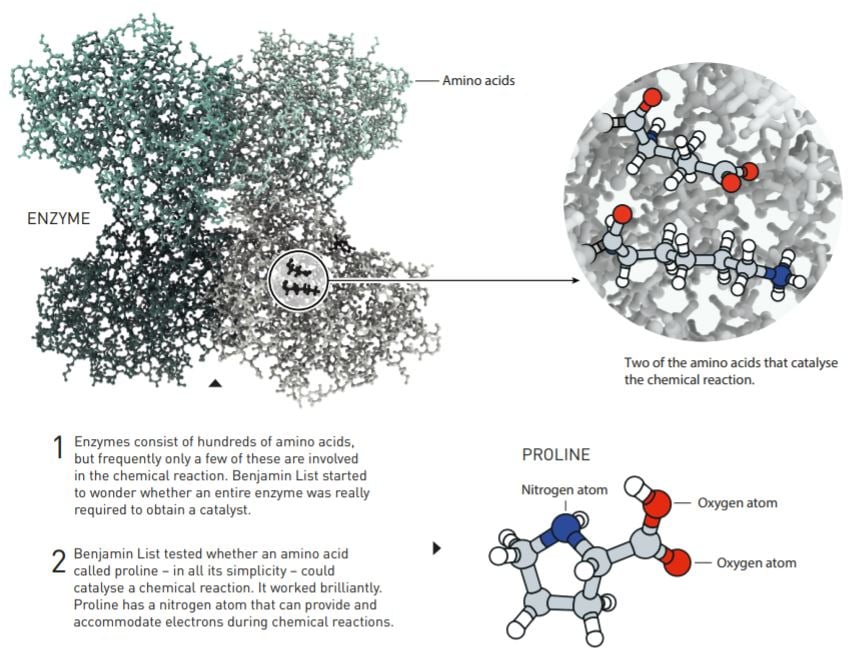
Benjamin List staked out his future
With his experiments, Benjamin List not only demonstrated that proline is an efficient catalyst, but also that this amino acid can drive asymmetric catalysis. Of the two possible mirror images, it was much more common for one of them to form than the other.
Unlike the researchers who had previously tested proline as a catalyst, Benjamin List understood the enormous potential it could have. Compared to both metals and enzymes, proline is a dream tool for chemists. It is a very simple, cheap and environmentally-friendly molecule. When he published his discovery in February 2000, List described asymmetric catalysis with organic molecules as a new concept with many opportunities: “The design and screening of these catalysts is one of our future aims”.
However, he was not alone in this. In a laboratory further north in California, David MacMillan was also working towards the same goal.
David MacMillan leaves sensitive metals behind…
Two years previously, David MacMillan had moved from Harvard to UC Berkeley. At Harvard he had worked on improving asymmetric catalysis using metals. This was an area which was attracting a lot of attention from researchers, but David MacMillan noted how the catalysts that were developed were rarely used in industry. He started to think about why, and assumed that the sensitive metals were quite simply too difficult and expensive to use. Achieving the oxygen-free and moisturefree conditions demanded by some metal catalysts is relatively simple in a laboratory, but conducting large-scale industrial manufacturing in such conditions is complicated.
His conclusion was that if the chemical tools he was developing were to be useful, he needed a rethink. So, when he moved to Berkeley, he left the metals behind.
…and develops a simpler form of catalyst
Instead, David MacMillan started to design simple organic molecules which – just like metals – could temporarily provide or accommodate electrons. Here, we need to define what organic molecules are – in brief, these are the molecules that build all living things. They have a stable framework of carbon atoms. Active chemical groups are attached to this carbon framework, and they often contain oxygen, nitrogen, sulphur or phosphorus.
Organic molecules thus consist of simple and common elements but, depending on how they are put together, they can have complex properties. David MacMillan’s knowledge of chemistry told him that for an organic molecule to catalyse the reaction he was interested in, it needed to be able to form an iminium ion. This contains a nitrogen atom, which has an inherent affinity for electrons.
He selected several organic molecules with the right properties, and then tested their ability to drive a Diels–Alder reaction, which chemists use to build rings of carbon atoms. Just as he had hoped and believed, it worked brilliantly. Some of the organic molecules were also excellent at asymmetric catalysis. Of two possible mirror images, one of them comprised more than 90 per cent of the product.

David MacMillan coins the term organocatalysis
When David MacMillan was ready to publish his results, he realised that the concept for catalysis he had discovered needed a name. The fact was that researchers had previously succeeded in catalysing chemical reactions using small organic molecules, but these were isolated examples and no one had realised that the method could be generalised.
David MacMillan wanted to find a term to describe the method so other researchers would understand that there were more organic catalysts to discover. His choice was organocatalysis.
In January 2000, just before Benjamin List published his discovery, David MacMillan submitted his manuscript for publication in a scientific journal. The introduction states: “Herein, we introduce a new strategy for organocatalysis that we expect will be amenable to a range of asymmetric transformations”.
The use of organocatalysis has boomed
Independently of each other, Benjamin List and David MacMillan had discovered an entirely new concept for catalysis. Since 2000, developments in this area can almost be likened to a gold rush, in which List and MacMillan maintain leading positions. They have designed multitudes of cheap and stable organocatalysts, which can be used to drive a huge variety of chemical reactions.
Not only do organocatalysts often consist of simple molecules, in some cases – just like nature’s enzymes – they can work on a conveyor belt. Previously, in chemical production processes it was necessary to isolate and purify each intermediate product, otherwise the volume of byproducts would be too great. This led to some of the substance being lost at every step of a chemical construction.
Organocatalysts are much more forgiving as, relatively often, several steps in a production process can be performed in an unbroken sequence. This is called a cascade reaction, which can considerably reduce waste in chemical manufacturing.
Strychnine synthesis now 7,000 times more efficient
One example of how organocatalysis has led to more efficient molecular constructions is the synthesis of the natural, and astoundingly complex, strychnine molecule. Many people will recognise strychnine from books by Agatha Christie, queen of the murder mystery. However, for chemists, strychnine is like a Rubik’s Cube: a challenge that you want to solve in as few steps as possible.
When strychnine was first synthesised, in 1952, it required 29 different chemical reactions and only 0.0009 per cent of the initial material formed strychnine. The rest was wasted.
In 2011, researchers were able to use organocatalysis and a cascade reaction to build strychnine in just 12 steps, and the production process was 7,000 times more efficient.
Organocatalysis is most important in pharmaceutical production
Organocatalysis has had a significant impact on pharmaceutical research, which frequently requires asymmetric catalysis. Until chemists could conduct asymmetric catalysis, many pharmaceuticals contained both mirror images of a molecule; one of these was active, while the other could sometimes have unwanted effects. A catastrophic example of this was the thalidomide scandal in the 1960s, in which one mirror image of the thalidomide pharmaceutical caused serious deformities in thousands of developing human embryos.
Using organocatalysis, researchers can now make large volumes of different asymmetric molecules relatively simply. For example, they can artificially produce potentially curative substances that can otherwise only be isolated in small amounts from rare plants or deep-sea organisms.
At pharmaceutical companies, the method is also used to streamline the production of existing pharmaceuticals. Examples of this include paroxetine, which is used to treat anxiety and depression, and the antiviral medication oseltamivir, which is used to treat respiratory infections.
Simple ideas are often the most difficult to imagine
It is possible to list thousands of examples of how organocatalysis is used – but why did no one come up with this simple, green and cheap concept for asymmetric catalysis earlier? This question has many answers. One is that the simple ideas are often the most difficult to imagine. Our view is obscured by strong preconceptions about how the world should work, such as the idea that only metals or enzymes can drive chemical reactions. Benjamin List and David MacMillan succeeded in seeing past these preconceptions to find an ingenious solution to a problem with which chemists had struggled for decades. Organocatalysts are thus bringing – right now – the greatest benefit to humankind.
Further reading
Additional information on this year’s prizes, including a scientific background in English, is available on the website of the Royal Swedish Academy of Sciences, www.kva.se, and at www.nobelprize.org, where you can watch video footage of the press conferences, the Nobel Lectures and more. Information on exhibitions and activities related to the Nobel Prizes and the Prize in Economic Sciences is available at www.nobelprizemuseum.se
The Royal Swedish Academy of Sciences has decided to award the Nobel Prize in Chemistry 2021 to
BENJAMIN LIST
Born 1968 in Frankfurt, Germany.
Ph.D. 1997 from Goethe University
Frankfurt, Germany. Director of the
Max-Planck-Institut für Kohlenforschung,
Mülheim an der Ruhr, Germany.
DAVID W.C. MACMILLAN
Born 1968 in Bellshill, UK. Ph.D. 1996
from University of California, Irvine, USA.
Professor at Princeton University, USA.
“for the development of asymmetric organocatalysis”
Science Editors: Peter Brzezinski, Peter Somfai, Johan Åqvist, the Nobel Committee for Chemisty
Text: Ann Fernholm
Translator: Clare Barnes
Illustrations: © Johan Jarnestad/The Royal Swedish Academy of Sciences
Editor: Sara Gustafsson
© The Royal Swedish Academy of Sciences
Play a game!
Chiral objects, like left and right hands, cannot be superimposed on its mirror image. Find other ‘chiral’ objects in this game.
Nobel Prizes and laureates
Six prizes were awarded for achievements that have conferred the greatest benefit to humankind. The 12 laureates' work and discoveries range from proteins' structures and machine learning to fighting for a world free of nuclear weapons.
See them all presented here.