Advanced information
Scientific Background:
Discovery of cancer therapy by inhibition of negative immune regulation
Scientific Background
Discovery of cancer therapy by inhibition of negative immune regulation
The 2018 Nobel Prize in Physiology or Medicine is awarded to James P. Allison and Tasuku Honjo for their discovery of cancer therapy by inhibition of negative immune regulation. Already 150 years ago attempts were made to use infectious agents to stimulate immune responses to cancer. Since then many seminal discoveries in the fields of basic immunology and tumor biology, including some awarded with the Nobel Prize, enabled a deeper understanding of such processes. In 1996, James P. Allison and coworkers used this accumulated knowledge to demonstrate that antibodies directed against a cell surface molecule on T cells, CTLA-4, is capable of unleashing an immune response, which cured mice from tumors. Prior to this, in the laboratory of Tasuku Honjo, a new molecule named PD-1 had been identified. Similar to CTLA-4, PD-1 also serves as a brake, which can prevent T cells from killing cancer cells. With the aid of biomedical companies Allison succeeded in developing the new concept with anti-CTLA-4 into clinical therapy for patients with advanced forms of melanoma. The use of antibodies directed against PD-1 and its ligand PD-L1 has now been approved for several cancer forms and this treatment is even more efficacious. The best clinical results have so far been obtained by combining treatment with anti-CTLA-4 and anti- PD-1. These antibodies are known as immune checkpoint inhibitors and the seminal discoveries by the two laureates have added a new pillar for the treatment of cancer.
Cancer
Cancer is a common term for a group of diseases caused by uncontrolled cell proliferation and migration. This results in abnormal growth of a tumor mass, first within an organ, then infiltrating adjacent tissues. Eventually, cancer cells can also colonize distant organs via blood or lymphatic vessels, so called metastases, causing morbidity and death. The symptoms, course and prognosis of the disease vary depending on the tissue origin. The risk of cancer increases with age and the remarkably longer life span of the world population contributes to cancer as an increasing problem. Life style and environmental factors add to this development, with smoking as the most important factor.
According to WHO, more than 18 million persons in the world are estimated to be diagnosed with cancer in 2018 (Global Cancer Observatory, 2018). Today, one in three will develop cancer in the economically more developed countries and it is estimated to be one in two in two decades. The number has increased steadily during the last 50 years. Within the same period the proportion of cured patients, as estimated by five-year survival, has increased from less than a third to more than two thirds. That said, these are average figures, which conceal major differences – between cancers in different organs, between earlier and more advanced forms of the disease in the same organ, and also between different countries. The occurrence of metastasis often implies that it is impossible to cure the disease, even if life can be prolonged by different treatments. In countries with less developed economy, a lower proportion of patients are diagnosed and treated, resulting in fewer cures and reduced average survival.
Scientific discoveries have paved the way for better understanding of the disease, as well as for novel treatments. Etiology and pathogenesis is complex, but all cancers display certain hallmarks (Hanahan & Weinberg, 2011). Some of these concern intrinsic cellular processes that regulate division, death, metabolism and migration;; others influence interactions in the tumor microenvironment with cells forming blood vessels or stroma, and cells of the immune system. Each of the hallmarks is influenced by multiple pathways, which have been rendered dysfunctional by alterations in the genes of cancer cells. Some of these genetic changes can be inherited via the germ line DNA, while the majority is acquired as mutations in somatic cells in processes influenced by a variety of external factors.
Several discoveries leading to today’s knowledge about cancer represent breakthroughs that have been awarded with The Nobel Prize in Physiology or Medicine. Those discoveries awarded have included infection as an etiological factor (e g Rous 1966, for tumor-inducing viruses;; zur Hauzen 2008, for Human Papilloma virus as a cause of cervical cancer);; and the relation between cellular and viral genes in pathogenesis (e g Baltimore, Dulbecco and Temin 1975, for integration of retroviral genetic information into DNA;; Bishop and Varmus 1989, for the cellular origin of viral oncogenes). In addition, novel therapies have also been awarded the Nobel Prize in Physiology or Medicine, e g Huggins 1966, for hormone treatment of prostate cancer;; Elion and Hitchings 1988, for novel principles leading to cytostatic drugs affecting the metabolism of nucleic acids, and Thomas 1990, for discoveries concerning bone marrow transplantation, used to treat certain blood cancers. These therapies were introduced in the latter half of the previous century to complement the traditional methods of surgery and radiotherapy. Additional Nobel Prizes, including in Chemistry, have awarded groundbreaking discoveries regarding basic cellular functions with relevance to cancer regarding DNA replication, cell cycle mechanisms, apoptosis, and genome integrity.
Despite this remarkable progress, the incidence of cancer is rising in most countries of the world, partly due to an increasing life span and improved diagnosis;; one out of three will develop the disease and one of 6 will die of cancer (Global Cancer Observatory, 2018). In 2018, the global mortality from cancer is estimated to surpass 9 million people. The scientific knowledge has led to programs for prevention and early diagnosis. However, even dramatically improved prevention programs will not solve the problem, since it is estimated that at least 50% of cases may be caused by accumulation of spontaneous DNA mutations occurring during normal ageing (Tomasetti et al., 2013). Novel types of treatment are strongly needed, and there has been a huge investment in basic research on normal and cancer cells, with the aim of acquiring basic knowledge as a platform for new therapeutic concepts.
Immunotherapy for tumors until 1995
Throughout history there are many accounts on tumors disappearing after infectious episodes, whereas the investigation of experimental infections as therapy for cancer patients dates back to the late 19th century. The basic concept behind such treatments has been the possibility that the infections stimulated the immune system, leading to an immune rejection of the tumor. Most well-known for studies in this field is the American surgeon William B. Coley, who reported on the treatment of malignant tumors by repeated “inoculation of erysipelas”, i.e. of live, cultured Streptococci, in the early 1890s (Coley 1891). However, the first attempts in this field were by German clinicians 150 years ago (Busch, 1868, Fehleisen, 1882). While infectious therapy was practiced in numerous tumor patients, the clinical outcome varied, causing disbelief within the medical community. Today, the concept is manifested in the form of alternating intradermal and intracavitary administration of Bacillus Calmette-Guerin to patients with bladder tumors (Morales et al 1976;; Alexandroff et al., 1999).
In the early 20th century, the mechanism underlying the effect of infectious agents on tumors was not understood, but the concept that immunity could influence tumor development was around. In the beginning of the last century Leo Loeb mentioned the possible role of immunity for the growth of experimentally transplanted tumors (Loeb, 1902). It was apparent that a basic understanding of the immune system was needed. Inbred mouse strains were established. Using this new possibility it was discovered that alleles within at the histocompatibility-2 (H-2) locus in the mouse were key determinants for tumor transplantation (Snell & Higgins, 1951) and George D. Snell became a Nobel laureate in 1980 for his work in this field. These groundbreaking discoveries paved the way for the understanding of how lymphocytes use molecules of the Major Histocompatibility Complex (MHC) to discriminate ‘self’ from ‘non-self’ and through this process can identify and eliminate infectious intruders. Related to this, the Nobel Prize in 1996 was awarded to Peter C. Doherty and Rolf M. Zinkernagel “for their discoveries concerning the specificity of the cell mediated immune defence”.
Another example from the early 20th century relates to the Nobel laureate Paul Ehrlich, who shared the 1908 Prize with Ilya Ilyich Mechnikov “in recognition of their work on immunity”. He discussed in depth the potential effects of natural and acquired immunity for cancer (Ehrlich, 1909). Sixty years later Sir Frank Macfarlane Burnet, who shared the 1960 Nobel Prize “for discovery of acquired immunological tolerance”, proposed that the immune system serves as a surveillance system for cancer (Burnet, 1970).
A sign of the belief in immunotherapy for tumors is that a very large part of the funding in the field of immunology has been directed towards cancer research. This led to a number of fundamental discoveries in tumor immunology, e g the demonstration of tumor-specific antigens (Klein & Klein, 1962) and their molecular nature (Lurquin et al., 1989), cytotoxic T cell killing of tumor cells (Brunner et al., 1968), tumor-infiltrating lymphocytes (Klein et al., 1977) and their role in immunotherapy (Rosenberg et al., 1986) and immunoselection and immunoediting during tumor progression (Dunn et al., 2002). Numerous reports on experimental animal studies demonstrated profound, beneficial effects of the immune system on tumor growth. However, translating these findings into clinical therapy failed almost without exception. Moreover, numerous case reports have described treatment effects on cancer patients related to immunotherapy, but also here, when such novel approaches were tested in controlled clinical studies, there were typically failures or logistical problems in translating the complicated protocols. There are a few examples of success, one being that allogeneic bone marrow transplants, apart from serving as a replacement of hematopoietic tissue, also cause a graft-versus-leukemia effect (Weiden et al., 1979).
Moreover, in the 1980s two different approaches using complex protocols involving culture of patients’ lymphocytes ex vivo were developed, one based on tumor-infiltrating lymphocytes (Rosenberg et al., 1990) and another on a gene therapy concept, namely the generation of chimeric antigen-receptor (CAR) T cells (re- viewed in June & Sadelain, 2018). In 2017, the US Food and Drug Administration, (FDA) and this year, the European Medicines Agency (EMA) approved autologous CAR-T cell transfer for the treatment of leukemia.
T cell activation and the concept of costimulation
T cells have been at the center stage in immunology, but up until the 1980s their antigen- recognizing receptor remained elusive, whereas the antigen-specific receptor on B cells was already well characterized. Thus, during their differentiation B cells were known to rearrange their immunoglobulin genes (Nobel Prize to Susumu Tonegawa in 1987), express the resulting immunoglobulin protein on their surface and secrete large amounts of it into body fluids. In 1975 the development of the monoclonal antibody technology by George J F Köhler and César Milstein, for which they were awarded the Nobel Prize in 1984, paved the way for the identification of novel cell surface markers. This technology became crucial not only for identifying key molecules on T cells, but also for the new treatment that this year’s Nobel Prize awards.
Major developments for the understanding of cellular adaptive immune responses took place in the 1980s. During this decade the T cell receptor (TCR) was identified and its interaction with MHC-associated peptides on antigen-presenting cells was revealed. The gene for interleukin-2, an important regulator of T cells, was cloned and the mechanism underlying the signaling of the TCR was beginning to be understood. It became clear that the interaction between the TCR and MHC antigen was insufficient for activation of T cells, leading to the concept of costimulation. The deciphering of the underlying mechanisms was an incremental process that took place during the 1980s and 1990s involving many laboratories all over the world.
The first cell surface molecule identified in TCR costimulation was CD28, which was originally discovered by a monoclonal antibody recognizing this protein on T cells and thymocytes (Hansen et al., 1980). CD28 was found to synergize with the TCR complex in the activation of T cells (Martin et al., 1986). Four years later a ligand for CD28 was identified as the B7 molecule (now known as CD80), which is expressed on antigen-presenting cells (Linsley et al., 1990). Lieping Chen, Peter Linsley and coworkers (Linsley et al., 1992) suggested a role for costimulation in tumor immunology by demonstrating that the transfer of the B7 gene to tumor cells caused rejection, as shown also by James P. Allison’s group (Townsend & Allison, 1993).
Identification of CTLA-4 as a negative regulator
In 1987, incidentally the same year as the CD28 gene was isolated, the cDNA for a T cell- expressed, CD28-related molecule, CTLA-4 (cytotoxic T lymphocyte antigen 4), also named CD152, was cloned in the laboratory of Pierre Golstein (Brunet et al., 1987). The function of CTLA-4 was unknown, but structurally both CD28 and CTLA-4 belong to the immunoglobulin superfamily. It is now known that CTLA-4 protein resides intracellularly in resting T cells, but translocates rapidly to the membrane after activation (Lindsten et al., 1993;; Linsley et al., 1996). In contrast to other T cells, it is constitutively expressed as a membrane protein in regulatory T cells (Takahashi et al., 2000). Linsley et al. (1991) used soluble CTLA-4-Ig to demonstrate that CTLA-4, similar to CD28, binds to B7, but with higher affinity. In the following years the generally held view was that CD28 and CTLA-4 acted in a similar, costimulatory way. However, in 1994, Jeffrey Bluestone, Craig Thompson and colleagues (Walunas et al.1994), and subsequently, Krummel & Allison (1995) came to the conclusion that CTLA-4 instead served as a negative regulator of T cell activation. This was initially interpreted as an intrinsic effect on the T cell, where CTLA-4 would induce negative signalling interfering with positive signals elicited by TCR and costimulatory receptors. Several alternative or additional explanations are discussed today. Both the above studies were possible through the generation of monoclonal antibodies directed against CTLA-4. Inactivation of the Ctla4 gene in mice, performed in the laboratories of Arlene
Sharpe and Tak Mak, confirmed and further substantiated its negative regulatory role, since these mice developed very severe autoimmune disease associated with proliferating T cells (Tivol et al., 1995;; Waterhouse et al., 1995). Soluble CTLA4-Ig was later used by Linsley and colleagues as a decoy to block costimulation, developing it into a drug, abatacept, for treatment of rheumatoid arthritis (Linsley et al., 1992).
Inhibition of tumor growth by antibodies against CTLA-4 in animals
While some investigators used this new knowledge to develop treatments for autoimmunity (Lenschow et al., 1992), Allison followed another path. In spite of all the previous failures, he intended to find a cure for cancer. He attempted to block the negative effects that CTLA-4 induced, thereby unleashing an immune response. In contrast to most other concepts in tumor immunology, there was no need to understand which antigens the T cells would recognize, and the strategy was not selective for a particular type of tumor, but was in essence universal. The first experiment was set up in his laboratory at the University of California, Berkeley in the end of 1994 with an immediate blinded repeat over the Christmas period. Mice that had been transplanted with tumors were treated with monoclonal antibodies against CTLA-4. The result was spectacular (Fig. 1 and Fig. 4), and eventually led to the seminal discovery that this treatment directed towards the immune system, had curative potential in tumor-bearing mice (Leach et al 1996). Thus, (1) the authors confirmed the hypothesis that blocking of CTLA-4 would strengthen the T cell anti-tumor response, (2) even pre-established tumors were found to be sensitive, (3) rejection was followed by durable tumor immunity and (4) two types of tumors were tested and both responded. One of the tumor cell lines studied was a colon carcinoma, used with as well as without transfection with the murine B7.1 (CD80) gene. Both cells responded to therapy with antibodies directed against CTLA-4.
This work represents the birth of a new concept for immunotherapy. Monoclonal antibodies with similar capacity to unleash responses against tumors are nowadays often referred to as immune checkpoint inhibitors (ICIs).
The Allison laboratory initially carried out a number of studies in other animal tumor models including, prostate cancer (Kwon et al., 1997), mammary tumor (Hurwitz et al., 1998) and melanoma (van Elsas et al., 1999). Some of these investigations included combined therapies such as the use of anti-CTLA-4 and the immunostimulatory cytokine, GM-CSF (granulocyte-macrophage colony stimulating factor). In a short period, Allison had thus proved that several different tumor types responded to the same treatment strategy. Given the many previous failures of immune therapies demonstrated in mice, the challenge remained to develop the concept into clinical practice – could this be achieved?

Figure 1. The discovery of James P. Allison and coworkers, utilizing the role of CTLA-4 as an inhibitor of activation and developing antibodies to release the brake. The graph shows the effect of anti-CTLA-4 treatment in tumor-bearing mice compared to controls.
The development of clinical immune checkpoint inhibitor therapy
Translating this research into the clinics became a long-term goal of Allison. At the time there was very limited interest from the pharmaceutical industry for a treatment based on the removal of the brakes of immune responses, without knowledge on which antigens were recognized on the tumor cells. Moreover, the severe autoimmune phenotype of mice lacking CTLA-4 posed a major risk for severe side effects. However, through perseverance Allison eventually managed to establish collaboration with Alan Korman from the small biotech company, Medarex, in which an acquired technology based on transgenic mice made it possible to generate human monoclonal antibodies. An anti-CTLA-4 IgG1 monoclonal antibody named MDX-010 was developed in 1999, later named ipilimumab (Wolchok et al., 2013). The company Bristol-Myers Squibb subsequently acquired Medarex and continued the clinical development. With these studies, Jedd Wolchok and Stephen Hodi together with scientists at Bristol-Myers Squibb Company advanced the clinical program for the study of the new ICI.
In the first-in-man study MDX-010 was given to 9 patients in a phase I clinical trial at a single dose of 3 mg/kg and a response was observed in some melanoma patients (Hodi et al., 2003). The same year complete regression was reported in another trial in some treated melanoma patients, while severe autoimmune side effects were also observed (Phan et al., 2003). The responses were frequently recorded later than for chemotherapy. A special feature was the observation that treatment initially could even increase the tumor volume, “pseudoprogression”, owing to the infiltration of immune cells, rather than reducing it immediately, as usually seen with chemo- or radiotherapy.
Additional clinical studies were carried out and the major breakthrough came from a phase III trial for the treatment of unresectable, metastatic melanoma showing a significantly increased overall survival (Hodi et al., 2010). This trial was also the basis for the subsequent approval of anti-CTLA-4 by the FDA and the EMA in 2011.
Discovery of the PD-1 receptor and its role in immune responses
The second discovery awarded by this year’s Nobel Prize also originated in basic, curiosity- driven research, not primarily oriented towards cancer. PD-1 (CD279) was identified and cloned by Tasuku Honjo’s group at Kyoto University in Japan already in the early 1990s (Ishida et al., 1992), during the explosive progress in the field of costimulation, but before Allison’s discovery of CTLA-4 inhibition to treat cancer. At the time, Honjo and colleagues assumed that PD-1, identified by subtractive hybridization to isolate mRNAs overexpressed in dying mouse cells, would be involved in pathways regulating apoptosis. Hence, they used the acronym PD (for Programmed Cell Death). The open reading frame predicted a protein with a transmembrane region, distantly related to the immunoglobulin gene superfamily. Follow-up studies reported the structure and chromosomal location of the gene (Shinohara et al., 1994), as well as the generation of a monoclonal antibody for PD-1 used to demonstrate expression on activated T- and B-cells as well as on immature thymocytes (Agata et al., 1996;; Nishimura et al., 1996).
The function of PD-1 remained elusive for many years. Honjo launched an ambitious program to fully understand its function, in which the generation of mice deficient for this molecule was central. The first “knock-out” mice on C57Bl genetic background only showed a mild, intriguing phenotype with some splenomegaly and augmented B cell proliferation (Nishimura et al., 1998). Late in life, the mice developed a lupus like disease Nishimura et al., 1999). It took almost 10 years from the first discovery before a distinct picture emerged. By then, additional knock-out strains on multiple genetic backgrounds had revealed different types of T cell driven autoimmune syndromes (Nishimura and Honjo, 2001;; Nishimura et al, 2001), i.e. a pattern resembling the CTLA-4 knock-out mice described above. Honjo concluded that PD-1, similar to CTLA-4, acts by negatively controlling immune responses. By then it had already emerged that PD-1 belongs to the extended CD28/CTLA-4 family of receptors, and that the cytoplasmic tail contains an essential “immunoreceptor tyrosine based switch motif (ITSM)” (Okazaki et al., 2001).
In parallel, Honjo and colleagues had engaged in a hunt for the ligand of PD-1. They identified it together with the groups of Gordon Freeman and Clive Wood (Freeman et al, 2000) and it was named PD-1 ligand (PD-L1). A human equivalent of the molecule had been described already the year before in the laboratory of Lieping Chen (Dong et al., 1999), in search for genes belonging to the B7 family of ligands. The identified molecule, B7-H1 was not a ligand for CD28 or CTLA-4 and binding to PD-1 was not investigated in this study. The Freeman et al. report (2000) was also the first to discuss the possibility that some tumors may use PD-L1 to inhibit an antitumor immune response, based on the observation that the molecule was expressed, not only by macrophages, dendritic cells and additional immune cells, but also by certain cancer cells. A second ligand for PD-1, PD-L2 (CD273), was identified soon thereafter, in further collaboration between the groups of Freeman, Honjo and Sharpe (Latchman et al., 2001).
PD-1 blockade as a treatment of cancer
The general concept that the PD-1/PD-L1 pathway might be involved in immune responses to tumors was first tested in two studies published in 2002, one from Chen’s laboratory (Dong et al 2002) and another from the Kyoto groups of Minato and Honjo, in collaboration (Iwai et al 2002). Both reports focused on PD-L1 molecules expressed on tumor cells, demonstrating that expression of this ligand could protect transformed cells from an immune attack in vivo, and that this could be reversed by antibodies to PD-L1. The paper by Iwai et al was the first to discuss possible synergistic effects in combination therapy based on blockade of the PD-1 and CTLA-4 pathway. The concept of a role of the PD-1/PD-L1 pathway in tumor immunology was now established (Carrera & Collins, 2002;; Greenwald et al., 2002;; Dong and Chen, 2003).
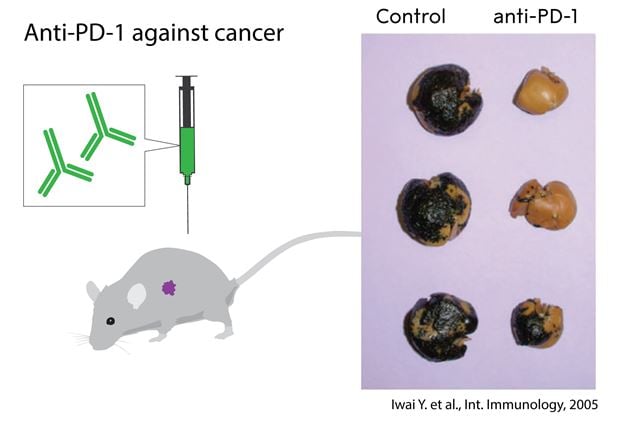
Figure 2. The discovery by Tasuku Honjo and coworkers, the identification of the PD-1 surface protein, recognizing its role as an inhibitor of activation and developing antibodies to release the brake. The graph shows the effect of anti-PD- 1 treatment in mice with metastasizing melanoma compared to untreated controls.
The first studies where an antibody to the receptor PD-1 was used to treat experimental cancer in mice were published in 2005 (Fig. 2 and Fig. 4). The paper from Honjo’s group presented several key conclusions that correctly anticipated the clinical pattern emerging today: anti-PD-1 treatment could a) induce immune responses, also to tumors that did not express detectable PD-L1 or PD-L2 b) be at least as (or even more) efficient as CTLA-4 therapy to treat tumors;; c) lead to less severe autoimmune side effects than CTLA-4 (Iwai et al, 2005). In parallel, Chen’s group used B7-H1/PD-L1 transfected cancer cells to demonstrate efficacy of antibody inhibition of PD-1 or PD-L1 in the treatment of tumors that expressed high levels of ligands (Hirano et al, 2005).
This development led to clinical studies based on PD-1 inhibition and Honjo and coworkers had filed a patent based on this concept. This was advanced by the company Ono Pharmaceuticals, which at an early stage joined forces with Bristol- Myers Squibb for the development of clinical grade antibodies directed against PD-1. The clinical trials of the PD-1 antibody nivolumab were led by Suzanne Topalian. The first phase I study, initiated in 2006, showed that the drug was well tolerated (Brahmer et al., 2010). Two years later, Topalian et al. reported the use of anti-PD-1 antibodies in 296 patients in a phase I study, indicating remarkable efficacy in treatment of advanced disease of different tumor types (Fig. 3). Objective responses were seen in 20-25% of patients with advanced non-small-cell-lung cancer (NSCLC), melanoma, or renal cancer, most of which were durable and some resulting in complete tumor regression (Topalian et al., 2012). In 14% of patients, severe adverse events, mainly pneumonitis, occurred. A phase I trial of another PD-1 antibody, pembrolizumab, developed by Merck (at the time called lambrolizumab), in 135 melanoma patients also showed very positive results, with clinical responses in 38% of patients, most of them durable and some of them observed in patients who had not responded (or ceased to respond) to anti-CTLA-4 treatment (Hamid et al., 2013). In parallel, several randomized phase III trials, where treatment with the PD-1 antibodies was compared to arms in which patients received standard chemotherapy, confirmed their efficacy and safety (Brahmer et al., 2015;; Borghaei et al., 2015;; Motzer et al., 2017). The first marketing and manufacturing approval was granted 2014 in Japan. This was followed by FDA approval through accelerated and breakthrough filing pathways, for two different anti PD-1 antibodies later the same year, pembrolizumab and nivolumab, for the treatment of unresectable or metastatic melanoma. The next year the approval was expanded to patients with metastatic squamous non-small cell lung cancer in March 2015 by FDA and EMA approved the use of PD-1 blockade in Europe for treatment of melanoma the same year.
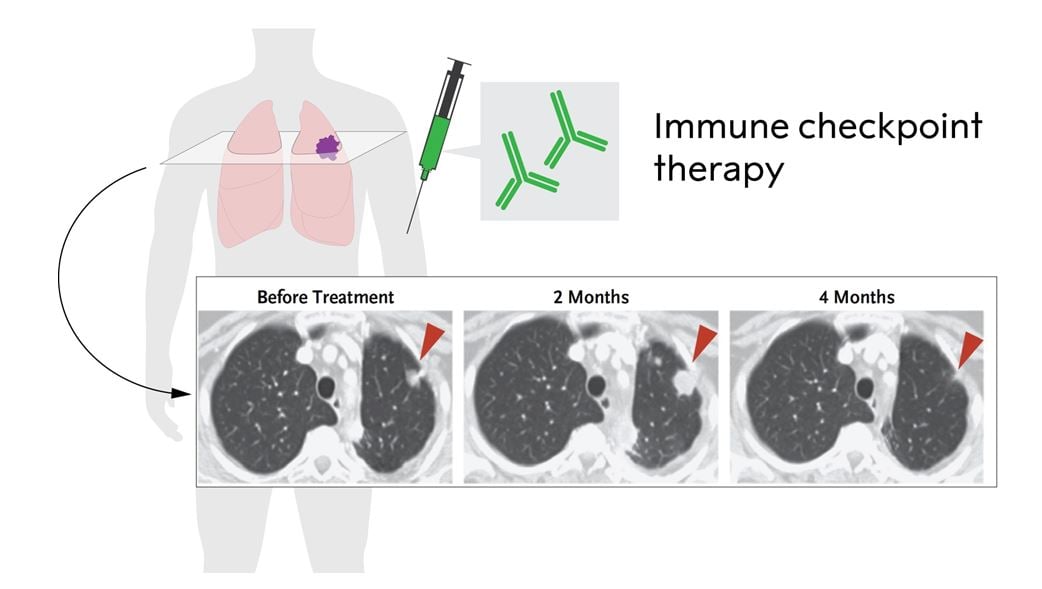
Figure 3. Immune checkpoint therapy in non-small cell lung cancer treated with anti-PD-1 (adopted from Topalian et al., 2012). Please note the pseudoprogression at 2 months due to infiltrating immune cells and the reduced tumor size after 4 months.
Cancer therapy using checkpoint inhibition today
More than fifteen years has passed since the first dose of anti-CTLA4 antibodies was given to a patient, while the first treatment with anti-PD-1 antibodies was administered twelve years ago. Today, there is a solid clinical experience, based not only on mono-treatment with each antibody, but also on combination treatment. The introduction of ICIs has dramatically changed the situation for patients with advanced, metastatic melanoma. Most of the responses are durable, and the curves that follow tumor-free survival show a plateau. In resected stage III and IV melanoma anti-PD-1 yielded significantly improved results as compared to anti-CTLA-4 treatment with ipililumab (Weber et al., 2017). Recurrence-free survival after 18 months of treatment with pembrolizumab in resected stage III melanoma was 71% (Eggermont, 2018). This is a spectacular development when considering metastatic disease of a solid tumor, which previously meant death within two years for most patients.
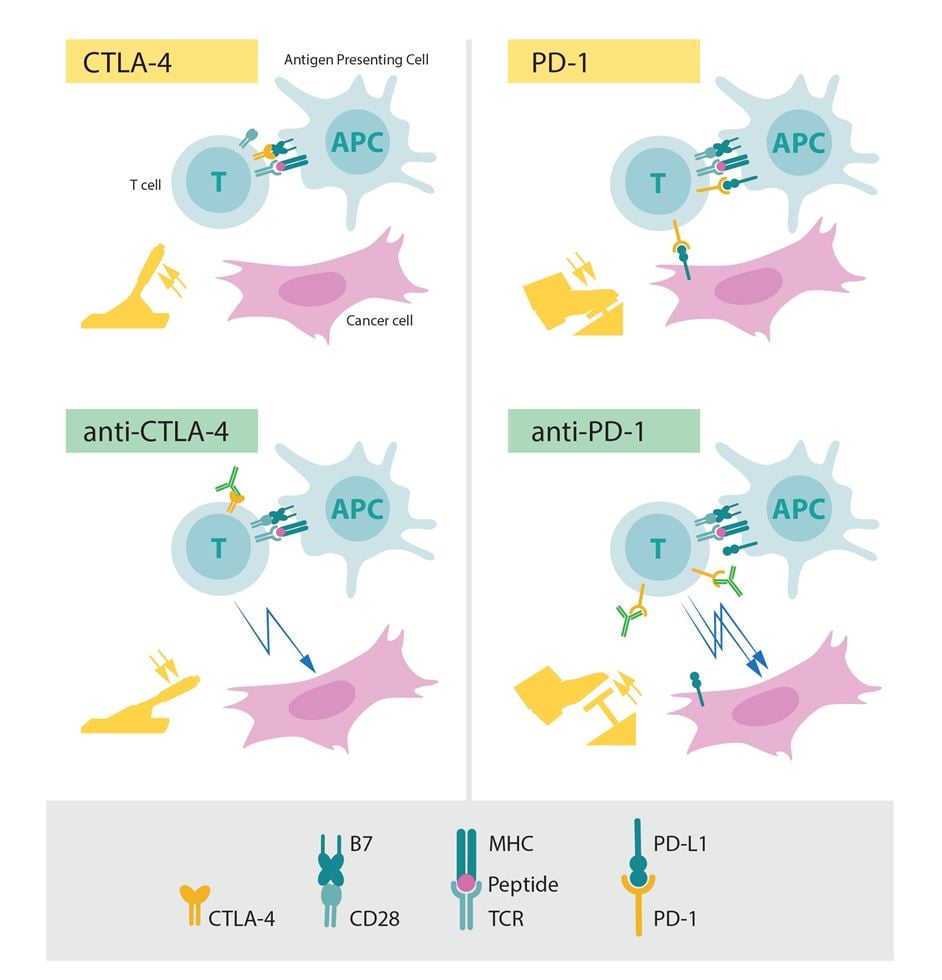
Figure 4. The role of CTLA-4 and PD-1 as inhibitors of activation (upper panels) and the effect of releasing the corresponding brakes using antibodies (lower panels).
While the outcome of multiple studies suggest that ICIs against PD-1 or its ligand, PD-L1, yield better treatment effects as compared to anti-CTLA-4, combined therapy with blockade of both CTLA-4 and PD-1 seems to induce an even stronger anti-tumor effect in both melanoma (Wolchock et al., 2013;; Wolchock et al., 2017), depicted in Fig. 5, and in renal cell carcinoma (Motzer et al., 2018), possibly because of different mechanisms of action. As expected, there are severe side effects in the form of autoimmunity, in particular during anti-CTLA-4 treatment (Eggermont et al., 2018;; Postow et al., 2018). Regardless of which therapy that is employed, immune-related adverse events occur at a high rate, as the immune system is no longer fully controlled, although with different clinical presentations. With inhibition of CTLA-4, severe colitis or endocrine dysfunction affecting the pituitary-adrenal axis are not uncommon, whereas thyroiditis shows a similar frequency in patients treated with anti-CTLA-4 or anti-PD- 1/PD-L1. Adrenal insufficiency whether caused by inflammation of the pituitary or destruction of the adrenal cortex can be life-threatening due to inability to produce endogenous cortisol, but can be easily treated with hydrocortisone supplementation, whereas e.g. pneumonitis may require aggressive immunomodulatory treatment with e.g. synthetic steroid or monoclonal antibody therapy directed against tumor necrosis factor (TNF). As with many cancer treatment modalities, the adverse reactions associated with immune checkpoint therapy can in some instances be fatal.
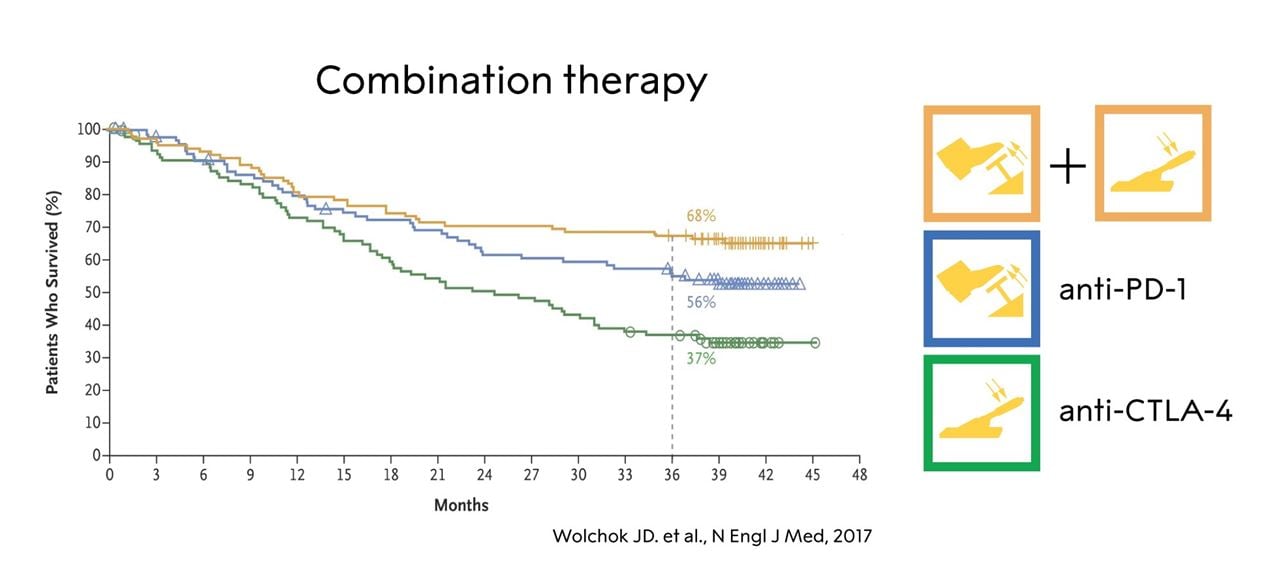
Figure 5. The effect of anti-CTLA-4, anti-PD-1 and combination therapy in a subgroup of patients with melanoma (adopted from Wolchock et al., 2017).
The ICIs have revolutionized the treatment of tumors, where there previously was precious little to offer, and while still many patients do not respond, there are numerous long-term survivors. Using other forms of treatment, durable recurrence-free survival is extremely rare among patients with certain tumors, such as advanced melanoma and NSCLC.
Currently very large numbers of studies for almost all cancer forms are being carried out worldwide and the development initiated by the study of CTLA-4 and PD-1 and its ligands is likely to continue. Promising results have been reported for anti-PD-1 treatment in several types of cancer, even if there is still limited information on cure and long-term survival. There are so far six anti-PD-1 or anti–PD-L1 antibodies approved by the FDA. The tumors that show the highest frequency of responses (50-90%) are Hodgkin´s lymphoma especially in patients with overexpression of PD-L1 and PD-L2 caused by gene amplification (Ansell et al., 2015), Merkel cell carcinoma of the skin, being of viral origin (Nghiem et al., 2016), in microsatellite-instability cancers of any origin having high mutational load from mismatch-repair deficiency (Le et al., 2017) and in desmoplasmic melanoma carrying numerous, UV-induced mutations (Eroglu et al., 2018). Incidentally, the anti PD-1 antibodies were the first class of agents granted approval by the FDA based on a genetic characteristic of the cancer rather on the organ or histological origin of the disease in 2016.
The development is likely to continue, with combination treatments – using different agents to release the negative regulation of immune responses, but also by combining them with strategies to activate or improve immune responses (e g vaccination, adoptive cell therapy) or simply combining them with existing modalities (surgery, chemotherapy, radiotherapy, hormone treatment and drugs targeting specific pathways) in adjuvant or neo-adjuvant protocols. Novel ICIs will undoubtedly add additional fuel into this development with numerous candidates in the pipeline of the biotech and pharmaceutical industry.
Mechanisms in the clinical response and how to predict it
It is so far clear that the common and general mechanism by which checkpoint therapy functions is via unleashing of the negative control of T cells, leading to stronger and more sustained immune response to the tumor. Immuno- histological and transcriptomic studies of biopsies indicate that a proportion of tumor infiltrating T cells, and signs of immune activation in the tumor prior to or during treatment, are associated with a favorable outcome (reviewed in Ribas & Wolchok, 2018). An analysis of 1535 advanced cancer patients treated with immune checkpoint inhibitors demonstrated that maximal heterozygosity at HLA class I loci improved overall survival, suggesting that antigen presentation to T cells plays a key role (Chowell et al., 2018). The molecular mechanisms of primary as well as acquired resistance of tumor cells to anti PD-1 therapy converge in alterations in the antigen presentation machinery (e.g. loss of b2-microglobulin, Zaretsky et al., 2016) and in the IFN-g receptor pathway controlling PD-L1 expression (Ribas & Wolchok, 2018).
The responses can include and be influenced by several downstream mechanisms, mediated also by other cells of the immune system. These cells and various molecules are intensively studied as biomarkers, and it should be emphasized that the critical effector mechanisms may vary between tumors, stage of disease and among patients depending on their genetic constitution as well as on their previous immune responses.
There are several interesting observations on how processes upstream of the initial T cell activation can correlate with the response of the patient. These processes include mutational load in the tumor, and, as a consequence, its expression of antigens, previous infections or vaccination, and the microenvironment and the microbiome in niches such as the colon or the skin (Snyder et al., 2014;; Rizvi et al., 2015;; Van Allen et al., 2015;; Le et al, 2017;; Mariathasan, 2018;; Tauriello et al., 2018;; Gopalakrishnan et al., 2018;; Routy et al., 2018).
The actual mechanisms whereby the antibodies interfere with negative regulation are also complex. It has been suggested that temporal and spatial differences between the CTLA-4 and PD-1 pathways, due to receptor expression kinetics and ligand expression pattern, lead to different and complementary roles in the immune response, and consequently, synergistic effects in combination therapy. It was initially assumed that anti-CTLA-4 antibodies worked by interfering with intrinsic T cell signaling and mainly acting in lymph nodes to prevent initial T cell activation. However, the importance of intrinsic signaling is debated, and later studies by several groups, including Allison’s, have provided evidence for at least four different mechanisms 1) Blockade of a direct inhibitory intracellular signaling pathway in effector T cells 2) Competition for, or sequestration of, activating ligands on antigen presenting cells 3) Interference with the function of regulatory T cells 4) Elimination of regulatory T cells. Note that the last mechanism may be exerted in the local tumor environment via antibody dependent cell mediated cytotoxicity.
Regarding PD-1, there is strong evidence that the antibodies act predominantly via T cell intrinsic signaling, mediated by the ITSM in the cytoplasmic tail of the molecule (Okazaki et al., 2001). Furthermore, a major part of the effect occurs intratumorally to counteract exhaustion of infiltrating T cells. Indeed, cancer cells frequently express PD-L1, and PD-L1 staining of tumor biopsies has therefore been examined extensively as a predictive marker. While there is a correlation between tumor cell expression of the ligand PD-L1 by immunohistochemistry and the likelihood of response to anti-PD-1, some patients (and mice) with undetectable PD-L1 on their tumors also respond (Brahmer et al., 2010;; Topalian et al., 2012), and effects in the lymph nodes are not excluded, nor are effects exerted via PD-1 expressed by regulatory T cells.
A crucial aspect in the future development is to improve understanding of these mechanisms, as well as the ones leading to adverse events. The identification of CTLA-4 and PD1/PD-L1 is only the beginning and additional molecules with similar functions are already being investigated. So far there is no single or combination of immune biomarkers that can predict the clinical response with certainty before or early in the treatment. There is intensive research going on, and the identification of key mechanisms and biomarkers is likely to improve clinical efficacy and safety as well as patient selection.
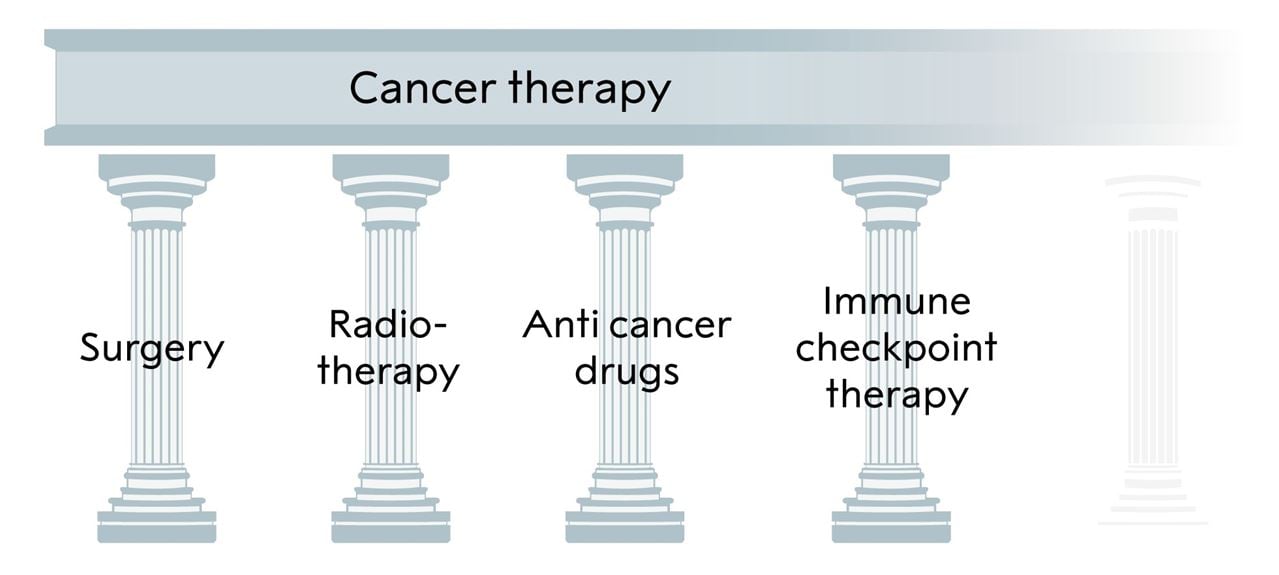
Figure 6. The three pillars of cancer treatment, all directed against the cancer cell, and the fourth, immune checkpoint inhibitor, based on unleashing an immune response against the tumor, added by Allison and Honjo.
Concluding remarks
This year’s Nobel Prize in Physiology or Medicine awards the discovery of a novel principle for tumor therapy based on removal of the brakes in T cells. This new form of immunotherapy unleashes a vigorous, and often durable, immune response directed against essentially any tumor already recognized by the immune system. Given the perhaps unprecedented research activities in the immune checkpoint field, it is likely that there will be major developments regarding this therapy at all levels. This demonstrates how influential the discoveries of Allison and Honjo have been. Their findings have conferred great benefit on mankind;; they add a new pillar to the existing cancer treatments (Fig. 6).
References
Agata Y, Kawasaki A, Nishimura H, Ishida Y, Tsubata T, Yagita H, Honjo T. Expression of the PD-1 antigen on the surface of stimulated mouse T and B lymphocytes. Int Immunol. 1996, 8(5):765-72.
Ansell SM, Lesokhin AM, Borrello I, Halwani A, Scott EC, Gutierrez M, Schuster SJ, Millenson MM, Cattry D, Freeman GJ, Rodig SJ, Chapuy B, Ligon AH, Zhu L, Grosso JF, Kim SY, Timmerman JM, Shipp MA,
Armand P. PD-1 blockade with nivolumab in relapsed or refractory Hodgkin’s lymphoma. N Engl J Med. 2015, 372(4):311-9.
Bailey MH, Tokheim C, Porta-Pardo E, Sengupta S, Bertrand D, Weerasinghe A, Colaprico A, Wendl MC, Kim J, Reardon B, Ng PK, Jeong KJ, Cao S, Wang Z, Gao J, Gao Q, Wang F, Liu EM, Mularoni L, Rubio- Perez C, Nagarajan N, Cortés-Ciriano I, Zhou DC, Liang WW, Hess JM, Yellapantula VD, Tamborero D, Gonzalez-Perez A, Suphavilai C, Ko JY, Khurana E, Park PJ, Van Allen EM, Liang H;; MC3 Working Group;; Cancer Genome Atlas Research Network, Lawrence MS, Godzik A, Lopez-Bigas N, Stuart J, Wheeler D, Getz G, Chen K, Lazar AJ, Mills GB, Karchin R, Ding L. Comprehensive Characterization of Cancer Driver Genes and Mutations. Cell. 2018, 173(2):371-385.e18.
Borghaei H, Paz-Ares L, Horn L, Spigel DR, Steins M, Ready NE, Chow LQ, Vokes EE, Felip E, Holgado E, Barlesi F, Kohlhäufl M, Arrieta O, Burgio MA, Fayette J, Lena H, Poddubskaya E, Gerber DE, Gettinger SN, Rudin CM, Rizvi N, Crinò L, Blumenschein GR Jr, Antonia SJ, Dorange C, Harbison CT, Graf Finckenstein F, Brahmer JR. Nivolumab versus Docetaxel in Advanced Nonsquamous Non-Small-Cell Lung Cancer. N Engl J Med. 2015, 373(17):1627-39.
Brahmer JR, Drake CG, Wollner I, Powderly JD, Picus J, Sharfman WH, Stankevich E, Pons A, Salay TM, McMiller TL, Gilson MM, Wang C, Selby M, Taube JM, Anders R, Chen L, Korman AJ, Pardoll DM, Lowy I, Topalian SL.Phase I study of single-agent anti-programmed death-1 (MDX-1106) in refractory solid tumors: safety, clinical activity, pharmacodynamics, and immunologic correlates. J Clin Oncol. 2010, 28(19):3167-75.
Brahmer JR, Rodríguez-Abreu D, Robinson AG, Hui R, Csőszi T, Fülöp A, Gottfried M, Peled N, Tafreshi A, Cuffe S, O’Brien M, Rao S, Hotta K, Zhang J, Lubiniecki GM, Deitz AC, Rangwala R, Reck M. Health- related quality-of-life results for pembrolizumab versus chemotherapy in advanced, PD-L1-positive NSCLC (KEYNOTE-024): a multicentre, international, randomised, open-label phase 3 trial. Lancet Oncol. 2017, 18(12):1600-1609.
Brunet JF, Denizot F, Luciani MF, Roux-Dosseto M, Suzan M, Mattei MG, Golstein P. A new member of the immunoglobulin superfamily--CTLA-4. Nature. 1987, 328(6127):267-70.
Brunner KT, Mauel J, Cerottini JC, Chapus B. Quantitative assay of the lytic action of immune lymphoid cells on 5″Cr-labelled allogeneic target cells in vitro;; inhibition by isoantibody and by drugs Immunology. 1968, 14:181-96.
Burnett FM. The concept of immunological surveillance. Prog Exp Tumor Res. 1970, 13:1-27.
Busch W. Aus der Sitzung der medicinischen Section vom 18. November 1867. Berliner klinische Wochenscr. 1868, 5:137-8.
Carreno BM, Collins M. The B7 family of ligands and its receptors: new pathways for costimulation and inhibition of immune responses. Annu Rev Immunol. 2002, 20:29-53.
Chowell D, Morris LGT, Grigg CM, Weber JK, Samstein RM, Makarov V, Kuo F, Kendall SM, Requena D, Riaz N, Greenbaum B, Carroll J, Garon E, Hyman DM, Zehir A, Solit D, Berger M, Zhou R, Rizvi NA, Chan TA. Patient HLA class I genotype influences cancer response to checkpoint blockade immunotherapy. Science. 2018, 359(6375):582-587.
Coley WB. Contribution to the knowledge of sarcoma. Ann Surg. 1891, 14(3):199-220.
Dong H, Chen L. B7-H1 pathway and its role in the evasion of tumor immunity. J Mol Med 2003, 81(5):281-7.
Dong H, Strome SE, Salomao DR, Tamura H, Hirano F, Flies DB, Roche PC, Lu J, Zhu G, Tamada K, Lennon VA, Celis E, Chen L. Tumor-associated B7-H1 promotes T-cell apoptosis: a potential mechanism of immune evasion. Nat Med. 2002, 8(8):793-800.
Dong H, Zhu G, Tamada K, Chen L. B7-H1, a third member of the B7 family, co-stimulates T-cell proliferation and interleukin-10 secretion. Nat Med. 1999, 5(12):1365-9.
Dunn GP, Bruce AT, Ikeda H, Old LJ, Schreiber RD. Cancer immunoediting: from immunosurveillance to tumor escape. Nat Immunol. 2002, 3:991-8.
Eggermont AMM, Robert C, Ribas A. The new era of adjuvant therapies for melanoma. Nat Rev Clin Oncol. 2018, 15(9):535-536.
Ehrlich P. Über den jetzigen Stand der Karzinomforschung. Nederlandsch Tijdschrift voor Geneeskunde 1909, 5:273-90.
Eroglu Z, Zaretsky JM, Hu-Lieskovan S, Kim DW, Algazi A, Johnson DB, Liniker E, Ben Kong, Munhoz R, Rapisuwon S, Gherardini PF, Chmielowski B, Wang X, Shintaku IP, Wei C, Sosman JA, Joseph RW, Postow MA, Carlino MS, Hwu WJ, Scolyer RA, Messina J, Cochran AJ, Long GV, Ribas A. High response rate to PD-1 blockade in desmoplastic melanomas. Nature. 2018, 553(7688):347-350
Fehleisen F. Ueber die Zuchtung der Erysipelkokken auf künstlichem Nährboden und ihre Uebertragsbarkeit auf den Menschen. Deutsche Med. Wochenschr. 1882, 8:553-4.
Forde PM, Chaft JE, Smith KN, Anagnostou V, Cottrell TR, Hellmann MD, Zahurak M, Yang SC, Jones DR, Broderick S, Battafarano RJ, Velez MJ, Rekhtman N, Olah Z, Naidoo J, Marrone KA, Verde F, Guo H, Zhang J, Caushi JX, Chan HY, Sidhom JW, Scharpf RB, White J, Gabrielson E, Wang H, Rosner GL, Rusch V, Wolchok JD, Merghoub T, Taube JM, Velculescu VE, Topalian SL, Brahmer JR, Pardoll DM. Neoadjuvant PD-1 Blockade in Resectable Lung Cancer. N Engl J Med. 2018, 378(21):1976-1986.
Freeman GJ, Long AJ, Iwai Y, Bourque K, Chernova T, Nishimura H, Fitz LJ, Malenkovich N, Okazaki T, Byrne MC, Horton HF, Fouser L, Carter L, Ling V, Bowman MR, Carreno BM, Collins M, Wood CR, Honjo T. Engagement of the PD-1 immunoinhibitory receptor by a novel B7 family member leads to negative regulation of lymphocyte activation. J Exp Med. 2000, 192(7):1027-34.
Gettinger S, Horn L, Jackman D, Spigel D, Antonia S, Hellmann M, Powderly J, Heist R, Sequist LV, Smith DC, Leming P, Geese WJ, Yoon D, Li A, Brahmer J. Five-Year Follow-Up of Nivolumab in Previously Treated Advanced Non-Small-Cell Lung Cancer: Results From the CA209-003 Study. J Clin Oncol. 2018, 36(17):1675-1684.
Global Cancer Observatory, 2018. http://gco.iarc.fr/
Greenwald RJ, Latchman YE, Sharpe AH. Negative co-receptors on lymphocytes. Curr Opin Immunol. 2002, 14(3):391-6.
Gopalakrishnan V, Spencer CN, Nezi L, Reuben A, Andrews MC, Karpinets TV, Prieto PA, Vicente D, Hoffman K, Wei SC, Cogdill AP, Zhao L, Hudgens CW, Hutchinson DS, Manzo T, Petaccia de Macedo M, Cotechini T, Kumar T, Chen WS, Reddy SM, Szczepaniak Sloane R, Galloway-Pena J, Jiang H, Chen PL, Shpall EJ, Rezvani K, Alousi AM, Chemaly RF, Shelburne S, Vence LM, Okhuysen PC, Jensen VB, Swennes AG, McAllister F, Marcelo Riquelme Sanchez E, Zhang Y, Le Chatelier E, Zitvogel L, Pons N, Austin-Breneman JL, Haydu LE, Burton EM, Gardner JM, Sirmans E, Hu J, Lazar AJ, Tsujikawa T, Diab A, Tawbi H, Glitza IC, Hwu WJ, Patel SP, Woodman SE, Amaria RN, Davies MA, Gershenwald JE, Hwu P, Lee JE, Zhang J, Coussens LM, Cooper ZA, Futreal PA, Daniel CR, Ajami NJ, Petrosino JF, Tetzlaff MT, Sharma P, Allison JP, Jenq RR, Wargo JA. Gut microbiome modulates response to anti-PD-1 immunotherapy in melanoma patients. Science. 2018, 359(6371):97-103.
Hanahan D, Weinberg RA. Hallmarks of cancer: the next generation. Cell. 2011, 144:646-74.
Hansen JA, Martin PJ, Nowinski RC. Monoclonal antibodies identifying a novel T-cell antigen and Ia antigens on human lymphocytes. Immunogenetics. 1980, 10:247-60.
Hellmann MD, Rizvi NA, Goldman JW, Gettinger SN, Borghaei H, Brahmer JR, Ready NE, Gerber DE, Chow LQ, Juergens RA, Shepherd FA, Laurie SA, Geese WJ, Agrawal S, Young TC, Li X, Antonia SJ. Nivolumab plus ipilimumab as first-line treatment for advanced non-small-cell lung cancer (CheckMate 012): results of an open-label, phase 1, multicohort study. Lancet Oncol. 2017, 8(1):31-41.
Herbst RS, Soria JC, Kowanetz M, Fine GD, Hamid O, Gordon MS, Sosman JA, McDermott DF, Powderly JD, Gettinger SN, Kohrt HE, Horn L, Lawrence DP, Rost S, Leabman M, Xiao Y, Mokatrin A, Koeppen H, Hegde PS, Mellman I, Chen DS, Hodi FS. Predictive correlates of response to the anti-PD-L1 antibody MPDL3280A in cancer patients. Nature. 2014, 515(7528):563-7.
Hirano F, Kaneko K, Tamura H, Dong H, Wang S, Ichikawa M, Rietz C, Flies DB, Lau JS, Zhu G, Tamada K, Chen L. Blockade of B7-H1 and PD-1 by monoclonal antibodies potentiates cancer therapeutic immunity. Cancer Res. 2005, 65(3):1089-96.
Hodi FS, Mihm MC, Soiffer RJ, Haluska FG, Butler M, Seiden MV, Davis T, Henry-Spires R, MacRae S, Willman A, Padera R, Jaklitsch MT, Shankar S, Chen TC, Korman A, Allison JP, Dranoff G. Biologic activity of cytotoxic T lymphocyte-associated antigen 4 antibody blockade in previously vaccinated metastatic melanoma and ovarian carcinoma patients. Proc Natl Acad Sci U S A. 2003, 100(8):4712-7.
Hodi FS, O’Day SJ, McDermott DF, Weber RW, Sosman JA, Haanen JB, Gonzalez R, Robert C, Schadendorf D, Hassel JC, Akerley W, van den Eertwegh AJ, Lutzky J, Lorigan P, Vaubel JM, Linette GP, Hogg D, Ottensmeier CH, Lebbé C, Peschel C, Quirt I, Clark JI, Wolchok JD, Weber JS, Tian J, Yellin MJ, Nichol GM, Hoos A, Urba WJ. . Improved survival with ipilimumab in patients with metastatic melanoma. N Engl J Med. 2010, 363(8):711-23. Erratum in: N Engl J Med. 2010, 363(13):1290.
Hurwitz AA, Yu TF, Leach DR, Allison JP. CTLA-4 blockade synergizes with tumor-derived granulocyte- macrophage colony-stimulating factor for treatment of an experimental mammary carcinoma. Proc Natl Acad Sci U S A. 1998, 95(17):10067-71.
Ishida Y, Agata Y, Shibahara K, Honjo T. Induced expression of PD-1, a novel member of the immunoglobulin gene superfamily, upon programmed cell death. EMBO J. 1992, 11(11):3887-95.
Iwai Y, Ishida M, Tanaka Y, Okazaki T, Honjo T, Minato N. Involvement of PD-L1 on tumor cells in the escape from host immune system and tumor immunotherapy by PD-L1 blockade. Proc Natl Acad Sci U S A. 2002, 99(19):12293-7.
Iwai Y, Terawaki S, Honjo T. PD-1 blockade inhibits hematogenous spread of poorly immunogenic tumor cells by enhanced recruitment of effector T cells. Int Immunol. 2005, 17(2):133-44.
June CH, Sadelain M. Chimeric antigen receptor therapy. N Engl J Med. 2018, 379(1):64-73.
Klein G, Klein E. Antigenic properties of other experimental tumors. Cold Spring Harb Symp Quant Biol 1962, 27: 463-70.
Klein E, Svedmyr E, Jondal M, Vanky F. Functional studies on tumor-infiltrating lymphocytes in man. Isr J Med Sci. 13:747-52, 1977
Krummel MF, Allison JP. CD28 and CTLA-4 have opposing effects on the response of T cells to stimulation. J Exp Med. 1995, 182(2):459-65.
Kwon ED, Hurwitz AA, Foster BA, Madias C, Feldhaus AL, Greenberg NM, Burg MB, Allison JP. Manipulation of T cell costimulatory and inhibitory signals for immunotherapy of prostate cancer. Proc Natl Acad Sci U S A. 1997, 94(15):8099-103.
Lamm DL, Thor DE, Harris SC, Reyna JA, Stogdill VD, Radwin HM.Bacillus Calmette-Guerin immunotherapy of superficial bladder cancer. J Urol. 1980, 124(1):38-40.
Latchman Y, Wood CR, Chernova T, Chaudhary D, Borde M, Chernova I, Iwai Y, Long AJ, Brown JA, Nunes R, Greenfield EA, Bourque K, Boussiotis VA, Carter LL, Carreno BM, Malenkovich N, Nishimura H, Okazaki T, Honjo T, Sharpe AH, Freeman GJ. PD-L2 is a second ligand for PD-1 and inhibits T cell activation. Nat Immunol. 2001, 2(3):261-8.
Le DT, Durham JN, Smith KN, Wang H, Bartlett BR, Aulakh LK, Lu S, Kemberling H, Wilt C, Luber BS, Wong F, Azad NS, Rucki AA, Laheru D, Donehower R, Zaheer A, Fisher GA, Crocenzi TS, Lee JJ, Greten TF, Duffy AG, Ciombor KK, Eyring AD, Lam BH, Joe A, Kang SP, Holdhoff M, Danilova L, Cope L, Meyer C, Zhou S, Goldberg RM, Armstrong DK, Bever KM, Fader AN, Taube J, Housseau F, Spetzler D, Xiao N, Pardoll DM, Papadopoulos N, Kinzler KW, Eshleman JR, Vogelstein B, Anders RA, Diaz LA Jr.
Mismatch repair deficiency predicts response of solid tumors to PD-1 blockade. Science. 2017, 357(6349):409-413.
Leach DR, Krummel MF, Allison JP. Enhancement of antitumor immunity by CTLA-4 blockade. Science. 1996, 271(5256):1734-6.
Leibson PJ. The regulation of lymphocyte activation by inhibitory receptors. Curr Opin Immunol. 2004, 16(3):328-36.
Lindsten T, Lee KP, Harris ES, Petryniak B, Craighead N, Reynolds PJ, Lombard DB, Freeman GJ, Nadler LM, Gray GS,Thompson CB, June CH. Characterization of CTLA-4 structure and expression on human T cells. J Immunol. 1993, 151(7):3489-99.
Linsley PS, Bradshaw J, Greene J, Peach R, Bennett KL, Mittler RS. Intracellular trafficking of CTLA-4 and focal localization towards sites of TCR engagement. Immunity. 1996, 4(6):535-43.
Linsley PS, Brady W, Urnes M, Grosmaire LS, Damle NK, Ledbetter JA. CTLA-4 is a second receptor for the B cell activation antigen B7. J Exp Med. 1991, 174(3):561-9
Linsley PS, Clark EA, Ledbetter JA. T-cell antigen CD28 mediates adhesion with B cells by interacting with activation antigen B7/BB-1. Proc Natl Acad Sci U S A. 1990, 87(13):5031-5.
Linsley PS, Wallace PM, Johnson J, Gibson MG, Greene JL, Ledbetter JA, Singh C, Tepper MA. Immunosuppression in vivo by a soluble form of the CTLA-4 T cell activation molecule. Science. 1992, 257:792-5.
Loeb L. Further Investigations in Transplantation of tumors. J Med Res. 1902, 8(1):44-73.
Lurquin C, Van Pel A, Mariamé B, De Plaen E, Szikora JP, Janssens C, Reddehase MJ, Lejeune J, Boon T. Structure of the gene of tum- transplantation antigen P91A: the mutated exon encodes a peptide recognized with Ld by cytolytic T cells. Ce 1989, 58:293-303.
Mariathasan S, Turley SJ, Nickles D, Castiglioni A, Yuen K, Wang Y, Kadel EE III, Koeppen H, Astarita JL, Cubas R, Jhunjhunwala S, Banchereau R, Yang Y, Guan Y, Chalouni C, Ziai J, Şenbabaoğlu Y, Santoro S, Sheinson D, Hung J, Giltnane JM, Pierce AA, Mesh K, Lianoglou S, Riegler J, Carano RAD, Eriksson P, Höglund M, Somarriba L, Halligan DL, van der Heijden MS, Loriot Y, Rosenberg JE, Fong L, Mellman I, Chen DS, Green M, Derleth C, Fine GD, Hegde PS, Bourgon R, Powles T. TGFβ attenuates tumour response to PD-L1 blockade by contributing to exclusion of T cells. Nature. 2018, 554(7693):544- 548.
Martin PJ, Ledbetter JA, Morishita Y, June CH, Beatty PG, Hansen JA. A 44 kilodalton cell surface homodimer regulates interleukin 2 production by activated human T lymphocytes. J Immunol. 1986, 136(9):3282-7.
Morales A, Eidinger D, Bruce AW. Intracavitary Bacillus Calmette-Guerin in the treatment of superficial bladder tumors. J Urol. 1976, 116(2):180-3.
Motzer RJ, Escudier B, McDermott DF, George S, Hammers HJ, Srinivas S, Tykodi SS, Sosman JA, Procopio G, Plimack ER, Castellano D, Choueiri TK, Gurney H, Donskov F, Bono P, Wagstaff J, Gauler TC, Ueda T, Tomita Y, Schutz FA, Kollmannsberger C, Larkin J, Ravaud A, Simon JS, Xu LA, Waxman IM, Sharma P;; CheckMate 025 Investigators.Nivolumab versus Everolimus in Advanced Renal-Cell Carcinoma. N Engl J Med. 2015, 373(19):1803-13.
Nghiem PT, Bhatia S, Lipson EJ, Kudchadkar RR, Miller NJ, Annamalai L, Berry S, Chartash EK, Daud A, Fling SP, Friedlander PA, Kluger HM, Kohrt HE, Lundgren L, Margolin K, Mitchell A, Olencki T, Pardoll DM, Reddy SA, Shantha EM, Sharfman WH, Sharon E, Shemanski LR, Shinohara MM, Sunshine JC, Taube JM, Thompson JA, Townson SM, Yearley JH, Topalian SL, Cheever MA. PD-1 Blockade with Pembrolizumab in Advanced Merkel-Cell Carcinoma. N Engl J Med. 2016, 374(26):2542-52
Nishimura H, Agata Y, Kawasaki A, Sato M, Imamura S, Minato N, Yagita H, Nakano T, Honjo T. Developmentally regulated expression of the PD-1 protein on the surface of double-negative (CD4-CD8-) thymocytes. Int Immunol. 1996, 8(5):773-80.
Nishimura H, Honjo T. PD-1: an inhibitory immunoreceptor involved in peripheral tolerance. Trends Immunol. 2001, 22(5):265-8.
Nishimura H, Minato N, Nakano T, Honjo T. Immunological studies on PD-1 deficient mice: implication of PD-1 as a negative regulator for B cell responses. Int Immunol. 1998, 10(10):1563-72.
Nishimura H, Nose M, Hiai H, Minato N, Honjo T. Development of lupus-like autoimmune diseases by disruption of the PD-1 gene encoding an ITIM motif-carrying immunoreceptor. Immunity. 1999, 11(2):141- 51.
Nishimura H, Okazaki T, Tanaka Y, Nakatani K, Hara M, Matsumori A, Sasayama S, Mizoguchi A, Hiai H, Minato N, Honjo T. Autoimmune dilated cardiomyopathy in PD-1 receptor-deficient mice. Science. 2001, 291(5502):319-22.
Okazaki T, Maeda A, Nishimura H, Kurosaki T, Honjo T. PD-1 immunoreceptor inhibits B cell receptor- mediated signaling by recruiting src homology 2-domain-containing tyrosine phosphatase 2 to phosphotyrosine. Proc Natl Acad Sci U S A. 2001, 98(24):13866-71.
Phan GQ, Yang JC, Sherry RM, Hwu P, Topalian SL, Schwartzentruber DJ, Restifo NP, Haworth LR, Seipp CA, Freezer LJ, Morton KE, Mavroukakis SA, Duray PH, Steinberg SM, Allison JP, Davis TA, Rosenberg SA. Cancer regression and autoimmunity induced by cytotoxic T lymphocyte-associated antigen 4 blockade in patients with metastatic melanoma. Proc Natl Acad Sci U S A. 2003, 100(14):8372- 7.
Postow MA, Sidlow R, Hellmann MD. Immune-Related Adverse Events Associated with Immune Checkpoint Blockade. N Engl J Med. 2018, 378(2):158-168.
Ribas A, Wolchok JD. Cancer immunotherapy using checkpoint blockade. Science. 2018, 359(6382):1350-5.
Rizvi NA, Hellmann MD, Snyder A, Kvistborg P, Makarov V, Havel JJ, Lee W, Yuan J, Wong P, Ho TS, Miller ML, Rekhtman N, Moreira AL, Ibrahim F, Bruggeman C, Gasmi B, Zappasodi R, Maeda Y, Sander C, Garon EB, Merghoub T, Wolchok JD, Schumacher TN, Chan TA. Cancer immunology. Mutational landscape determines sensitivity to PD-1 blockade in non-small cell lung cancer. Science. 2015, 348(6230):124-8.
Robert C, Ribas A, Wolchok JD, Hodi FS, Hamid O, Kefford R, Weber JS, Joshua AM, Hwu WJ, Gangadhar TC, Patnaik A, Dronca R, Zarour H, Joseph RW, Boasberg P, Chmielowski B, Mateus C, Postow MA, Gergich K, Elassaiss-Schaap J, Li XN, Iannone R, Ebbinghaus SW, Kang SP, Daud A. Anti- programmed-death-receptor-1 treatment with pembrolizumab in ipilimumab-refractory advanced melanoma: a randomised dose-comparison cohort of a phase 1 trial. Lancet. 2014, 384(9948):1109-17.
Rodig SJ, Gusenleitner D, Jackson DG, Gjini E, Giobbie-Hurder A, Jin C, Chang H, Lovitch SB, Horak C, Weber JS, Weirather JL, Wolchok JD, Postow MA, Pavlick AC, Chesney J, Hodi FS. MHC proteins confer differential sensitivity to CTLA-4 and PD-1 blockade in untreated metastatic melanoma. Sci Transl Med. 2018, 18;;10(450).
Rosenberg SA, Aebersold P, Cornetta K, Kasid A, Morgan RA, Moen R, Karson EM, Lotze MT, Yang JC, Topalian SL, Merino MJ, Culver K, Miller D, Blaese RM, Anderson WF. Gene transfer into humans-- immunotherapy of patients with advanced melanoma, using tumor-infiltrating lymphocytes modified by retroviral gene transduction. N Engl J Med. 1990, 323(9):570-8.
Rosenberg SA, Spiess P, Lafreniere R A new approach to the adoptive immunotherapy of cancer with tumor-infiltrating lymphocytes. Science. 1986, 233:1318-21.
Routy B, Le Chatelier E, Derosa L, Duong CPM, Alou MT, Daillère R, Fluckiger A, Messaoudene M, Rauber C, Roberti MP, Fidelle M, Flament C, Poirier-Colame V, Opolon P, Klein C, Iribarren K, Mondragón L, Jacquelot N, Qu B, Ferrere G, Clémenson C, Mezquita L, Masip JR, Naltet C, Brosseau S, Kaderbhai C, Richard C, Rizvi H, Levenez F, Galleron N, Quinquis B, Pons N, Ryffel B, Minard-Colin V, Gonin P, Soria JC, Deutsch E, Loriot Y, Ghiringhelli F, Zalcman G, Goldwasser F, Escudier B, Hellmann MD, Eggermont A, Raoult D, Albiges L, Kroemer G, Zitvogel L. Gut microbiome influences efficacy of PD- 1-based immunotherapy against epithelial tumors. Science. 2018, 359(6371):91-97
Schachter J, Ribas A, Long GV, Arance A, Grob JJ, Mortier L, Daud A, Carlino MS, McNeil C, Lotem M, Larkin J, Lorigan P, Neyns B, Blank C, Petrella TM, Hamid O, Zhou H, Ebbinghaus S, Ibrahim N, Robert Pembrolizumab versus ipilimumab for advanced melanoma: final overall survival results of a multicentre, randomised, open-label phase 3 study (KEYNOTE-006). Lancet. 2017, 390(10105):1853- 1862.
Shinohara T, Taniwaki M, Ishida Y, Kawaichi M, Honjo T. Structure and chromosomal localization of the human PD-1 gene (PDCD1). Genomics. 1994, 23(3):704-6.
Snyder A, Makarov V, Merghoub T, Yuan J, Zaretsky JM, Desrichard A, Walsh LA, Postow MA, Wong P, Ho TS, Hollmann TJ, Bruggeman C, Kannan K, Li Y, Elipenahli C, Liu C, Harbison CT, Wang L, Ribas A, Wolchok JD, Chan TA. Genetic basis for clinical response to CTLA-4 blockade in melanoma. N Engl J Med. 2014, 371(23):2189-2199.
Takahashi T, Tagami T, Yamazaki S, Uede T, Shimizu J, Sakaguchi N, Mak TW, Sakaguchi S. Immunologic self-tolerance maintained by CD25(+)CD4(+) regulatory T cells constitutively expressing cytotoxic T lymphocyte-associated antigen 4. J Exp Med. 2000, 192(2):303-10.
Tauriello DVF, Palomo-Ponce S, Stork D, Berenguer-Llergo A, Badia-Ramentol J, Iglesias M, Sevillano M, Ibiza S, Cañellas A, Hernando-Momblona X, Byrom D, Matarin JA, Calon A, Rivas EI, Nebreda AR, Riera A, Attolini CS, Batlle E. TGFβ drives immune evasion in genetically reconstituted colon cancer metastasis. Nature. 2018, 554(7693):538-543.
Tivol EA, Borriello F, Schweitzer AN, Lynch WP, Bluestone JA, Sharpe AH. Loss of CTLA-4 leads to massive lymphoproliferation and fatal multiorgan tissue destruction, revealing a critical negative regulatory role of CTLA-4. Immunity. 1995, 3(5):541-7.
Tomasetti C, Vogelstein B, Parmigiani G. Half or more of the somatic mutations in cancers of self- renewing tissues originate prior to tumor initiation. Proc Natl Acad Sci U S A. 2013, 110(6):1999-2004.
Topalian SL, Hodi FS, Brahmer JR, Gettinger SN, Smith DC, McDermott DF, Powderly JD, Carvajal RD, Sosman JA, Atkins MB, Leming PD, Spigel DR, Antonia SJ, Horn L, Drake CG, Pardoll DM, Chen L, Sharfman WH, Anders RA, Taube JM, McMiller TL, Xu H, Korman AJ, Jure-Kunkel M, Agrawal S, McDonald D, Kollia GD, Gupta A, Wigginton JM, Sznol M. Safety, activity, and immune correlates of anti- PD-1 antibody in cancer. N Engl J Med. 2012, 366(26):2443-54.
Townsend SE, Allison JP. Tumor rejection after direct costimulation of CD8+ T cells by B7-transfected melanoma cells. Science. 1993, 259(5093):368-70.
Walunas TL, Lenschow DJ, Bakker CY, Linsley PS, Freeman GJ, Green JM, Thompson CB, Bluestone JA. CTLA-4 can function as a negative regulator of T cell activation. Immunity. 1994, 1(5):405-13.
Van Allen EM, Miao D, Schilling B, Shukla SA, Blank C, Zimmer L, Sucker A, Hillen U, Foppen MHG, Goldinger SM, Utikal J, Hassel JC, Weide B, Kaehler KC, Loquai C, Mohr P, Gutzmer R, Dummer R, Gabriel S, Wu CJ, Schadendorf D, Garraway LA. Genomic correlates of response to CTLA-4 blockade in metastatic melanoma. Science. 2015, 350(6257):207-211.
van Elsas A, Hurwitz AA, Allison JP. Combination immunotherapy of B16 melanoma using anti-cytotoxic T lymphocyte-associated antigen 4 (CTLA-4) and granulocyte/macrophage colony-stimulating factor (GM- CSF)-producing vaccines induces rejection of subcutaneous and metastatic tumors accompanied by autoimmune depigmentation. J Exp Med. 1999, 190(3):355-66.
Waterhouse P, Penninger JM, Timms E, Wakeham A, Shahinian A, Lee KP, Thompson CB, Griesser H, Mak TW. Lymphoproliferative disorders with early lethality in mice deficient in Ctla-4. Science. 1995, 270(5238):985-8.
Weber J, Mandala M, Del Vecchio M, Gogas HJ, Arance AM, Cowey CL, Dalle S, Schenker M, Chiarion- Sileni V, Marquez-Rodas I, Grob JJ, Butler MO, Middleton MR, Maio M, Atkinson V, Queirolo P, Gonzalez R, Kudchadkar RR, Smylie M, Meyer N, Mortier L, Atkins MB, Long GV, Bhatia S, Lebbé C, Rutkowski P, Yokota K, Yamazaki N, Kim TM, de Pril V, Sabater J, Qureshi A, Larkin J, Ascierto PA;; CheckMate 238 Collaborators. Adjuvant Nivolumab versus Ipilimumab in Resected Stage III or IV Melanoma. N Engl J Med. 2017, 377(19):1824-1835.
Wolchok JD, Chiarion-Sileni V, Gonzalez R, Rutkowski P, Grob JJ, Cowey CL, Lao CD, Wagstaff J, Schadendorf D, Ferrucci PF, Smylie M, Dummer R, Hill A, Hogg D, Haanen J, Carlino MS, Bechter O, Maio M, Marquez-Rodas I, Guidoboni M, McArthur G, Lebbé C, Ascierto PA, Long GV, Cebon J, Sosman J, Postow MA, Callahan MK, Walker D, Rollin L, Bhore R, Hodi FS, Larkin J. Overall Survival with Combined Nivolumab and Ipilimumab in Advanced Melanoma. N Engl J Med. 2017, 377(14):1345-56.
Wolchok JD, Hodi FS, Weber JS, Allison JP, Urba WJ, Robert C, O’Day SJ, Hoos A, Humphrey R, Berman DM, Lonberg N, Korman AJ. Development of ipilimumab: a novel immunotherapeutic approach for the treatment of advanced melanoma. Ann N Y Acad Sci. 2013, 1291:1-13.
Wolchok JD, Kluger H, Callahan MK, Postow MA, Rizvi NA, Lesokhin AM, Segal NH, Ariyan CE, Gordon RA, Reed K, Burke MM, Caldwell A, Kronenberg SA, Agunwamba BU, Zhang X, Lowy I, Inzunza HD, Feely W, Horak CE, Hong Q, Korman AJ, Wigginton JM, Gupta A, Sznol M. Nivolumab plus ipilimumab in advanced melanoma. N Engl J Med. 2013, 369(2):122-33.
Zaretsky JM, Garcia-Diaz A, Shin DS, Escuin-Ordinas H, Hugo W, Hu-Lieskovan S, Torrejon DY, Abril- Rodriguez G, Sandoval S, Barthly L, Saco J, Homet Moreno B, Mezzadra R, Chmielowski B, Ruchalski K, Shintaku IP, Sanchez PJ, Puig-Saus C, Cherry G, Seja E, Kong X, Pang J, Berent-Maoz B, Comin- Anduix B, Graeber TG, Tumeh PC, Schumacher TN, Lo RS, Ribas A. Mutations Associated with Acquired Resistance to PD-1 Blockade in Melanoma. N Engl J Med. 2016, 375(9):819-29.
C.I. Edvard Smith1, Rikard Holmdahl2, Olle Kämpe3 & Klas Kärre4
1) Professor of Molecular Genetics
2) Professor of Medical Inflammation Research
3)Professor of Clinical Endocrinology
4) Professor of Molecular Immunology
Adjunct Members of the Nobel Committee and Members of the Nobel Assembly
Karolinska Institutet, Stockholm, September 30, 2018
Correspondence: [email protected]
Illustrations: Mattias Karlén
Nobel Prizes and laureates
Six prizes were awarded for achievements that have conferred the greatest benefit to humankind. The 12 laureates' work and discoveries range from proteins' structures and machine learning to fighting for a world free of nuclear weapons.
See them all presented here.